Future Optics: Complex devices make photonics more efficient: Interview with Hui Cao
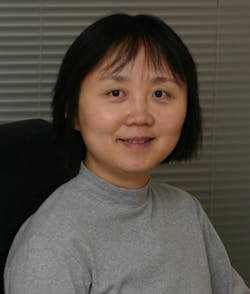
OSA: How did you get involved with optics?
Hui Cao: When I was very young, my father, a professor of physics at Peking University, asked me what travels fastest and furthest. I thought maybe a train or plane, but he said it is light. I was intrigued, and wanted to study optics to learn why light is so special. I studied quantum optics with my father for my bachelor's thesis. Then, I came to the U.S. in 1990—first to work on x-ray lasers with Szymon Suckewer at Princeton University, and then to Stanford University to study quantum optics with Yoshihisa Yamamoto.
OSA: What brought you here and why did you stay?
HC: I came here 26 years ago for graduate education, and I really liked the emphasis on independent thinking in the U.S. system. Students asked professors many questions and even argued with them. That helped me to develop my own interests, and do research that I find exciting and important rather than just following others. Right after I got my PhD from Stanford in 1997, Northwestern offered me an assistant professorship, so I decided to stay. I had fantastic collaborators and colleagues, and I spent 10 years there until I moved to Yale in 2008.
OSA: What led to your work on random lasers?
HC: I did my PhD study on semiconductor microcavity quantum electrodynamics and related devices-after moving to Northwestern, I wanted to do something different. I started collaborating with Robert P. Chang in Materials Science and Engineering to study the optical properties of zinc oxide (ZnO), a wide bandgap semiconductor that emits in the near-ultraviolet and has large optical nonlinearities. There was much interest in making lasers with ZnO, but it was hard to cleave or etch ZnO to make conventional laser cavities. I accidentally observed lasing when I measured the fluorescence from ZnO polycrystalline films. I was puzzled because there were no obvious cavities to support lasing. Eventually, I figured out that random scattering from the ZnO grains was providing the feedback for lasing. It was a surprise, as I was used to the laser cavities made of regular, ordered structures. The random laser is much easier to fabricate than a conventional laser.
OSA: What have we learned about random lasers and where are they going?
HC: Random lasers are complex, nonlinear, open systems, and their studies cross the fields of laser and mesoscopic physics, nonlinear dynamics, and quantum chaos. Also, their distinct characteristic leads to unique applications. For example, we found that their low spatial coherence could have important application in full-field imaging. The high spatial coherence of conventional lasers often produces artifacts such as speckle, while random lasers with low spatial coherence enable speckle-free imaging. Lamps and LEDs have been widely used as illumination sources for microscopes and projectors because of their low spatial coherence, but random lasers are much brighter sources that are more suitable for imaging in lossy or high-scattering environments such as biological tissue, or imaging transient processes.
The physical understanding we obtained from the studies on random lasers also led us to design and fabricate more practical sources for speckle-free, full-field imaging, namely the electrically pumped semiconductor laser that had low spatial coherence. It was based on a chaotic microcavity in which the ray dynamics is chaotic—one example is a circular disk with one flat side forming a D shape. The fabrication of chaotic microcavity lasers on chips was much easier than the electrically pumped random lasers and more suitable for mass production.
I have been working with Michael Choma at the Yale School of Medicine on developing light sources for massive parallel confocal microscopy and optical coherence tomography. We designed a laser system that can shift between high and low spatial coherence with little power loss. Such a system allows the acquisition of two types of microscopy images—one image is free of speckle and reveals the structure of the object, while the other image is full of speckle and the temporal fluctuation of speckle can tell the motion in the object. As an initial demonstration of the system's potential biomedical applications, we used it to image the blood flow and miniscule structural changes of a tadpole embryo's beating heart.
OSA: How did you get into nanophotonics?
HC: I started exploring wave optics after finishing my PhD in quantum optics. Unlike quantum optics, wave optics is a rather old field of physics. But in the last two decades, there have been rapid advances and breakthroughs in wave optics. Thanks to modern nanofabrication, complex nanostructures can be fabricated and display unusual optical properties, such as photonic crystals and metamaterials.
We have been working on complex photonic structures that are neither completely ordered, like photonic crystals, nor completely disordered. Ordered systems typically have just one spatial frequency, but disordered systems have many more and exhibit richer phenomena. Systems in between the two display a wide range of behaviors, giving us a lot of room to play.
Nature shows us how the interplay between order and disorder affects optical properties. One example is structural color, and there are two ways to generate color by nanostructures. Long-range periodic nanostructures generate iridescent colors that vary with angle. However, nanostructures with only short-range order can generate colors that barely change with angle, which are used by animals such as birds and butterflies. This shows what partially ordered structures can do that cannot be done by completely ordered structures. It's amazing how nature can make many complex photonic nanostructures by self-assembly in mass quantity, and there is a lot for us to learn and mimic.
One advantage of complex nanophotonic structures is that they provide many degrees of freedom to control optical phenomena, which may lead to multifunctional photonic devices. Another frontier is exploring the imaginary part of permittivity or permeability, which represent optical gain and loss. So far, the studies on photonic crystals and metamaterials aimed to manipulate only the real part of permittivity or permeability. Combining the two may open up possibilities for novel photonic devices.
OSA: What training do students need?
HC: I think interdisciplinary training is important for preparing students for the emerging world. With the rapid growth of computer power, students need to learn both hardware and software—for example, computational imaging and compressive sensing are getting popular now. Many years ago when I learned lens design, the goal was to make a perfect lens that can image one plane as clearly as possible. But now, aberration is intentionally induced to the lens so that multiple planes can be imaged simultaneously. Although the raw image looks smeared, efficient algorithms have been developed to recover multiple images of different planes. In other words, more information can be obtained from a "poor" lens than a "good" lens. I see a trend that will be important in the future is to combine hardware and software to make more efficient photonic devices and systems.
HUI CAO is a professor of applied physics and of physics at Yale University, and a fellow of The Optical Society and of the American Physical Society. She studies both the fundamental physics and applications of complex photonic materials and nanophotonic devices.