Scientists in the Nanotechnology Group at The Swiss Federal Institute of Technology Zurich (Zurich, Switzerland) have developed a tunable diffraction grating composed of soft electroactive polymer (EAP) actuators—also called “artificial muscles”—that achieves a grating-period change of 32%, or an improvement of more than a factor of 150 compared with existing analog tunable diffraction gratings that use hard materials.1 By combining this grating with a white-light source, it is possible to develop a wavelength-adjustable luminous source that can reproduce the entire range of visible colors to create low-cost color displays.
When illuminating a tunable grating with white light, an observer viewing a reflection from the grating will see different colors depending on the grating period. The grating period is tuned by changing the voltage (strain) applied to the grating, which is mounted on a dielectric elastomer actuator.
To fabricate this electrically tunable diffraction grating, an acrylic elastomer film is prestrained by a factor of 300% × 300%, which results in a film thickness of 62.5 µm. The film is mounted on a 3 × 3 mm holder and carbon-black electrodes are contact-printed on the film. A 20-µm-thick elastomer film is then spin-coated onto a holographic diffraction-grating master (1000 lines/mm) and the resulting uncured grating bonded to the acrylic elastomer film with electrodes. After curing, the grating master is peeled from the elastomer film, which remains bonded to the elastomer actuator. A 6-nm-thick gold layer is then evaporated onto the grating to increase reflectivity.
When applying a voltage between the two electrodes of the grating, charges build up at the elastomer-electrode interfaces and an electric-field pressure is created. This pressure induces stresses that result in a material strain that causes the elastomer to compress in thickness and elongate in the planar directions, changing the grating period. When the voltage is increased from 0 V up to 4.5 kV, a grating period change of 32% is observed, which is a factor of 152 better than the 0.21% strain reported for gratings that use stiff piezoelectric actuators.
The scientists illuminated the grating with a 532 nm laser and studied the change in the first-order diffraction angle as a function of applied voltage. For voltages up to 3.7 kV, the diffracted light kept its Gaussian shape and the first-order diffraction angle change was 118 mrad, a significant improvement over the 486 µrad change observed using piezoelectric analog gratings. In addition, stability tests performed by ramping the voltage from 0 to 4.5 kV for 30,000 cycles did not degrade the grating reflectivity or cause peeling of the gold layer.
To demonstrate the color display concept, collimated white light from a 150 W tungsten-halogen lamp with a spot size of 0.5 mm was directed at an incidence angle of 0.46 rad onto the center of the grating. A starting wavelength of 446 nm was recorded with 0 V applied. As the voltage increased, the images, recorded on a CCD as a function of voltage, clearly showed the color changes from blue to green, then to yellow and red (see figure). The measured observations compared favorably to the theoretical values, except for voltages above 4 kV due to an increase of the elastic modulus that was not taken into account in the theoretical curve.
“The technical reason for using artificial muscles for changing the grating period is their large strain,” says researcher Andreas Stemmer. “Furthermore, replicating a grating (hard material) into soft silicone material is a very precise and low-cost process.”
REFERENCE
1. M. Aschwanden and A. Stemmer, Optics Lett. 31(17) 2610 (Sept. 1, 2006).
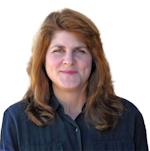
Gail Overton | Senior Editor (2004-2020)
Gail has more than 30 years of engineering, marketing, product management, and editorial experience in the photonics and optical communications industry. Before joining the staff at Laser Focus World in 2004, she held many product management and product marketing roles in the fiber-optics industry, most notably at Hughes (El Segundo, CA), GTE Labs (Waltham, MA), Corning (Corning, NY), Photon Kinetics (Beaverton, OR), and Newport Corporation (Irvine, CA). During her marketing career, Gail published articles in WDM Solutions and Sensors magazine and traveled internationally to conduct product and sales training. Gail received her BS degree in physics, with an emphasis in optics, from San Diego State University in San Diego, CA in May 1986.