Optoacoustics unveils thermodynamic surprise: Negative pressure within liquids
A project driven entirely out of curiosity by researchers at the Max Planck Institute for the Science of Light (MPL) and the Leibniz Institute of Photonic Technology (IPHT) in Germany recently led to the discovery of negative pressure within liquids.
Pressure is a force exerted per unit area that acts perpendicular to a surface of a solid, liquid, or gas. Under normal conditions, the numerical value of pressure is always positive.
But liquids are oddballs and come with a peculiar quirk: they can exist in a metastable state corresponding to a negative pressure value. Within this metastable state, even the tiniest external influence can trigger the system to collapse into another state.
Think of the influence of negative pressure on a liquid as envisioned with the laws of thermodynamics: while the volume of the liquid decreases, it’s retained within the glass fiber capillary by adhesive forces, which enables it to stretch like a rubberband.
“We initially set out to determine whether optoacoustics could help monitor thermodynamic behavior within completely sealed liquid-core optical fibers,” says Birgit Stiller, head of the quantum optoacoustics research group at MPL. “But when our measurements showed weird behavior and trends, it forced us to rethink our assumptions and theory.”
Metastable state of liquids with negative pressure
In an attempt to understand the weird results and the metastable state of liquids with negative pressure, Stiller and colleagues (see Fig. 1) combined optical and acoustic waves to measure thermodynamic states (see video).
“Brillouin-Mandelstam scattering is the interaction of two optical waves and one acoustic wave,” says Stiller. “The latter is very sensitive to temperature, pressure, and strain changes—and is therefore predestined for thermodynamic measurements.”
In case you caught the “Brillouin-Mandelstam scattering,” the researchers added “Mandelstam” as a nod of respect to finally acknowledge the work of Leonid Mandelstam, a Russian physicist who, sadly, was overlooked for his discovery of the combinational scattering of light in 1928 (which carries C. V. Raman’s name, although Mandelstam and Grigory Landsberg reported observing it a week earlier). Others within the photonics community are starting to add this nod to Mandelstam’s contributions as well.
To access different thermodynamic regimes, Stiller’s colleagues Mario Chemnitz and Markus Schmidt at IPHT in Jena pioneered a tiny and easy-to-use optical platform. “Without it, we wouldn’t be able to reach the different thermodynamic regimes—it’s a true and beneficial collaboration between my group at MPL and groups at IPHT,” Stiller says.
How does Chemnitz and Schmidt’s optical platform work? It encapsulates nanoliters of a liquid inside a fully enclosed fiber to enable both highly positive and negative pressures. And the interaction of optical and acoustic waves within the liquid allows researchers to measure the influence of pressure and temperature for different states of the liquid.
As you may guess, extreme safety precautions are necessary when measuring metastable states—especially when toxic liquids are involved. The researchers used carbon disulfide, which is a toxic liquid, and previous measurement setups to generate and determine negative pressures required significant lab space and could pose a disturbance to the system in a metastable state.
Instead, the group’s compact method “allows us to gain a deeper understanding of the thermodynamic dependencies within a unique fiber-based system,” says Alexandra Popp, a postgraduate member of Stiller’s group.
Surprises!
Optoacoustic interactions “offer huge potential, not only for signal processing, light storage, and microwave photonics but also to explore new routes in combination with other research fields,” says Stiller. “But be ready for unconventional findings—while being careful with your assumptions.”
Discovering negative pressure surprised the group. “The trend of the acoustic frequency clearly indicated a linear decrease that would lead to the assumption of negative pressure, but because we’re nonlinear quantum optics researchers we weren’t sure about this assumption,” says Stiller. “Interpreting our experimental results helped us learn much more about thermodynamics.”
Observations of the negative pressure regime “became abundantly clear when looking at the frequency of the sound waves,” says Andreas Geilen, a Ph.D. student working with Stiller.
Stiller’s group is still working on “challenges like reaching weird thermodynamic regimes within our liquid-core fibers,” she says. “These fibers require a special design and getting measurements might be more complicated.”
Liquid-based fiber sensors and quantum optics
Stiller expects their combination of optoacoustic measurements with tightly sealed capillary fibers to enable new discoveries via monitoring chemical reactions within toxic liquids for otherwise difficult-to-explore materials and microreactors—and to open up hard-to-access areas of thermodynamics.
Another one of her team’s discoveries was that the sensitivity of the liquid-core fibers within the isobaric regime is 7x higher than standard optical fibers, which may enable new liquid-based fiber sensors. Typical standard fibers change their acoustic frequency by ~1 MHz/°C, but for this type of fiber it’s ~7 MHz for 1°C—which would make it an ideal sensor choice for short sensing lengths (dozen of meters).
“Phenomena difficult to explore with ordinary and established methods can become unexpectedly accessible when new measurement methods are combined with novel platforms,” says Stiller.
The sound waves used by the researchers can detect temperature, pressure, and strain changes very sensitively along an optical fiber. Spatially resolved measurements are also possible, which means sound waves can provide an image of what’s happening inside the optical fiber at centimeter-scale resolution along its length.
Typical optoacoustic measurements provide an integrated image of the entire fiber length, but there are distributed fiber sensing methods that can capture a detailed picture of what’s happening every centimeter in the optical fiber.
The method Stiller’s group is using is inspired by radar (see Fig. 2). A broadband optical signal is sent into both ends of the fiber. In the middle of the setup, a correlation peak appears where the correlation between both waves is always the same. But everywhere else the optical waves behave randomly. This interaction of the optical correlation peak with localized acoustic waves gives the researchers information about how the acoustic waves change over a short distance of centimeters—it’s known as Brillouin optical correlation domain analysis.
“My group is also interested in quantum optics, so there’s a good chance this platform will provide a great playground for our quantum signal-processing ideas,” says Stiller. “We plan to now explore even weirder thermodynamic regimes and are ready for surprises—probably more for us, not so much for the thermodynamics researchers.”
FURTHER READING
A. Geilen et al., Nat. Phys. (2023); https://doi.org/10.1038/s41567-023-02205-1.
Related Articles
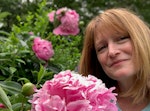
Sally Cole Johnson | Editor in Chief
Sally Cole Johnson, Laser Focus World’s editor in chief, is a science and technology journalist who specializes in physics and semiconductors. She wrote for the American Institute of Physics for more than 15 years, complexity for the Santa Fe Institute, and theoretical physics and neuroscience for the Kavli Foundation.