Mid-IR Lasers: Dysprosium mid-IR fiber laser generates picosecond pulses beyond 3 µm with rediscovered frequency shifting method
Extending short-pulse fiber laser technology to longer wavelengths is a significant challenge that must be addressed to enable new photonic applications such as laser surgery, countermeasures, and breath analysis. In the mid-infrared (mid-IR) region, erbium (Er)- and holmium (Ho)-doped fluoride glasses have been used as gain media to create 2.7–2.9 µm fiber lasers with picosecond pulses using mode-locking saturable absorbers. However, achieving ultrashort-pulsed fiber lasing at wavelengths beyond 3 µm has been unsuccessful because of material limitations.
Recognizing the demand for mid-IR short-pulse sources, researchers at Macquarie University (Sydney, Australia) have succeeded in developing a 33 ps pulsed fiber laser tunable from 2.97 to 3.30 µm using a dysprosium-doped gain fiber and acousto-optic tunable filter (AOTF) in a frequency-shifted feedback (FSF) configuration resurrected from the early days of laser development.1 The researchers say this is the longest-wavelength, broadest-tunability mode-locked mid-IR fiber laser demonstrated to date.
Rediscovering FSF
Conventional mode-locked lasers use cavity modulators (saturable absorbers) that preferentially enable pulsed over continuous-wave (CW) operation through a series of round trips within the fiber cavity that establish phase coherence. But an FSF fiber laser is very different: it includes a cavity frequency shifter, so the wavelength of the cavity light is shifted with each round trip. This frequency shift destabilizes the buildup of longitudinal mode structure and, at low pump powers, leads to incoherent emission since generated light repeatably grows from spontaneous emission and then is swept outside the gain bandwidth by the frequency shifter after multiple round trips. This results in a noisy temporal output that lacks coherence.
Nonlinear effects, however, convert this output into a coherent picosecond pulsed state. As pump power is increased, the intensity of cavity light grows, leading to self-phase modulation and spectral broadening (arising from the intrinsic Kerr nonlinearity of the fiber), which then balances the frequency-shifting effect. This balance prevents the cavity light from being swept outside the gain bandwidth, yielding coherent emission. Effectively, the balancing mechanism preferentially favors the generation of high-intensity waveforms—a train of short pulses.
This FSF technique originated in the 1960s wherein moving-cavity mirrors introduced a Doppler frequency shift that led to phase-locking lasers, which was later extended to fiber lasers in the early 1990s. It has since received very little attention, however, as semiconductor saturable absorbers emerged as the dominant method of pulse generation in the near-IR. But at longer mid-IR wavelengths where saturable absorbers are not well developed, the FSF technique could be very useful for next-generation pulsed-source development.
Dysprosium-doped ZBLAN
The physical setup for the FSF fiber laser consists of an Er-doped zirconium barium lanthanum aluminum sodium fluoride (ZBLAN) laser or Er:ZBLAN laser that pumps a length of dysprosium-doped ZBLAN (Dy:ZBLAN) optical fiber (see figure). The researchers note that dysprosium is a relatively new gain material for fiber lasers, offering significantly longer wavelength emission than Er and Ho. The cavity has a dichroic mirror at the pump output (gain fiber input) and an AOTF with gold mirror at the output of the gain fiber. The AOTF operates using traveling acoustic waves and it is the Doppler shift that these waves impart that causes the frequency shift.
As explained previously, FSF produces a pulsed output with an approximate 33 ps pulse duration. By varying the applied RF frequency to the AOTF, the filter’s center wavelength can be varied, tuning the laser from 2.97 to 3.30 μm. Stable picosecond pulses with up to 2.7 nJ can be generated at every wavelength in this range.
“This pulsed operation was actually discovered by accident,” says Robert Woodward, research fellow at Macquarie University. “We were initially surprised to see pulses generated while investigating the use of an AOTF, but after referring back to historic laser literature and performing detailed simulations, we discovered that FSF could explain this.” The wavelengths achieved cover absorption fingerprints for many important molecules, and the researchers are investigating ammonia gas sensing applications. Woodward concludes, “The combination of dysprosium fiber with FSF is an exciting step to overcome current material limitations that have previously held back mid-IR fiber laser technology.”
REFERENCE
1. R. I. Woodward, M. R. Majewski, and S. D. Jackson, “Mode-locked dysprosium fiber laser: picosecond pulse generation from 2.97 to 3.30 µm,” accepted for publication in APL Photonics, 3, 11 (2018).
About the Author
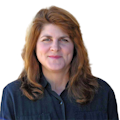
Gail Overton
Senior Editor (2004-2020)
Gail has more than 30 years of engineering, marketing, product management, and editorial experience in the photonics and optical communications industry. Before joining the staff at Laser Focus World in 2004, she held many product management and product marketing roles in the fiber-optics industry, most notably at Hughes (El Segundo, CA), GTE Labs (Waltham, MA), Corning (Corning, NY), Photon Kinetics (Beaverton, OR), and Newport Corporation (Irvine, CA). During her marketing career, Gail published articles in WDM Solutions and Sensors magazine and traveled internationally to conduct product and sales training. Gail received her BS degree in physics, with an emphasis in optics, from San Diego State University in San Diego, CA in May 1986.