PHOTONIC FRONTIERS: SEMICONDUCTOR UV LASERS: Materials are a tough challenge for ultraviolet diode lasers
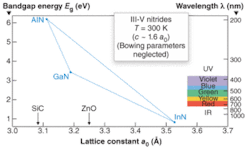
Aluminum gallium nitride can emit light at wavelengths close to 200 nm, but developers need another breakthrough in materials before they can push semiconductor diode lasers into the deep ultraviolet.
The 1990s saw the dramatic breakthrough of the blue diode laser and the mood was optimistic that diode lasers could be pushed to even shorter wavelengths. Judging from bandgaps alone, nitride-based III-V semiconductors offered the possibility of wavelengths as short as about 200 nm.
Yet that promise has proved hard to realize in this decade, at least for electrically driven diode lasers. Optical pumping has leapfrogged to shorter wavelengths, largely because it circumvents key limitations of semiconductor materials, but it is not considered practical for most potential applications. Light-emitting diodes also have reached deep into the ultraviolet because their operating conditions are much less demanding and they look promising for applications that don’t require laser light or high intensity. But electrically driven diode lasers remain stuck in the near-ultraviolet (near-UV)–the shortest reported wavelength has decreased just 1 nm in the past four years, although the latest progress raises new hopes.
The problem is materials, as it often has been for diode-laser development. The gallium-indium-nitride system that works so well for blue diode lasers runs out of bandgap in the near-UV. Pushing to shorter wavelengths requires adding aluminum to the mix, and growing good-quality semiconductors from the new blend is proving difficult. The electrical resistance of p-doped blends is troublingly high. Some developers are turning to LEDs as better bets for shorter wavelengths.
Near-UV diode lasers
At the start of the 1990s, even blue diode lasers seemed beyond reach. Semiconductor developers knew gallium indium nitride (GaInN) had the proper direct bandgap, but were stymied by poor material quality and the inability to make p-type material. That changed when Shuji Nakamura, then at Nichia (Anan, Tokushima-ken, Japan) tamed the problems with GaInN and opened the door to the blue, violet, and near-UV.
Three-five nitride compounds span a wide range of bandgap energies (see Fig. 1). The binary compounds and their bandgaps can be expressed both in energy and in wavelength (see table). Boron nitride also has a direct bandgap, but it’s about the same size as that of AlN, and the compound has not been doped successfully, so it’s not listed here.1Most nitride lasers are now made with emission at 405 nm (violet) for use in Blu-Ray optical disk players but the Laser Focus World Buyers Guide lists commercial diode lasers with emission from 375 to 445 nm. Developers have demonstrated GaInN laser diodes in the laboratory at wavelengths from 362 to 488 nm, with higher gallium fractions producing shorter wavelengths, ultimately limited to 362 nm by the bandgap of pure GaN.
Moving to shorter wavelengths requires adding aluminum to the semiconductor and the difficulties increase rapidly at shorter wavelengths. The small lattice constant of AlN produces tensile stress, leading to cracking in aluminum-rich layers in a diode. Growing the whole diode structure on an AlN substrate can help, but AlN itself is hard to grow– the only successful technique is limited to 1 cm wafers and is not suitable for mass production, wrote Ulrich Schwarz of the University of Regensburg (Regensburg, Germany) in the September issue of Nature Photonics.2 In addition, aluminum is prone to forming small crystallites that cause strain and dislocations in the semiconductor.
“Only a handful of laboratories have demonstrated laser diodes below 362 nm,” says Michael Kneissl of the Technical University of Berlin (Berlin, Germany). All but the most recent of those demonstrations have required adding indium to the semiconductor, forming the quaternary compound AlGaInN. The indium increases the quantum efficiency, but also reduces the bandgap energy.An indium-free UV laser
In September, Harumasa Yoshida and colleagues from the Central Research Laboratories of Hamamatsu Photonics (Hamamatsu, Japan) reported a small but important step forward: a multiple-quantum-well AlGaN laser diode emitting at 342.3 nm that contains no indium (see Fig. 2).3 In that device layers of GaN and Al0.3Ga0.7N with low dislocation density served as a buffer between the sapphire substrate and the diode layers. The active layer contained multiple quantum wells of Al0.04Ga0.96N surrounded by Al0.14Ga0.86N. The guiding layers above and below the active layer consisted of the same higher-aluminum compound.
The researchers reported a laser threshold of 390 mA, equivalent to 8.7 kA/cm2, when exciting their laser with 10 ns pulses at a 5 kHz repetition rate. Increasing the drive current above threshold caused line narrowing and decreased the wavelength, with the maximum 512 mA drive producing 16 mW output at 342.3 nm, with 0.3 nm linewidth.
That wavelength was only a small step beyond the previous record of 343 nm reported by Cree Inc. (Durham, NC) for a laser grown on silicon carbide.4 But both Kneissl and Schwartz hailed Hamamatsu for demonstrating an indium-free laser that points the way toward making shorter-wavelength devices.
More challenges in reducing resistance
The race is far from over. Kneissl says the next big challenge for short-wavelength diode lasers is to reliably generate a continuous-wave beam. He says reaching that goal will require both reducing dislocation densities to a million per square centimeter, and reducing internal resistance to limit heating of the laser.
The large bandgap needed for ultraviolet emission contributes to the conductivity problem. “Would you consider a material with a 6.2 eV bandgap–larger than that of diamond–a semiconductor?” Schwarz wrote. The biggest challenge is the p-type material. The magnesium dopant has a 0.25 eV activation energy in GaN, and that value increases linearly with the bandgap energy. The Hamamatsu laser needed a 25 V forward bias to overcome the device resistance at threshold, so it had to dissipate 10 W of heat during the drive pulses–too much power to dissipate during continuous-wave operation.
Yoshida said that Hamamatsu would try to reduce diode resistance as a next step toward developing a higher-performance device for continuous-wave operation. Other challenges include reducing dislocation density and pushing to shorter wavelengths. But they are optimistic that their demonstration points the way to shorter-wavelength diode lasers.
Alternative materials
The dark horse in semiconductor ultraviolet lasers is the zinc oxide family of II-VI semiconductors. Pure ZnO has a bandgap of 3.3 eV, only slightly smaller than GaN, and the bandgap can be increased by adding magnesium oxide, which has a 7.9 eV bandgap. The material has attractive properties including higher radiation tolerance than GaN compounds, according to Henry White, a professor at the University of Missouri (Columbia, MO). A company he helped found, MOXtronics (Columbia, MO), has demonstrated ultraviolet ZnO LEDs, a task that required producing both p- and n-doped material.
Zinc oxide has also attracted attention because granules can serve as scatterers and as light emitters in random lasers, in which feedback comes from scattering within a material rather than from cavity mirrors. Most experiments have pumped the random-laser material optically, but Siu Fung Yu of Nanyang Technological University (Singapore) has demonstrated electrical pumping of a p-i-n diode laser.5 That is potentially attractive because it avoids the need to cut or polish facets on ZnO.
Yet ZnO has its problems, including doping as well as a better understanding of III-V compounds. “Nitrides are at least a decade ahead on the learning curve to make viable devices and the entire technological infrastructure is well established,” says Kneissl.
UV LEDs and optical pumping
Competition is also coming from LEDs. “A lot of progress is being made in deep-ultraviolet LEDs,” says Noble Johnson of the Palo Alto Research Center (Palo Alto, CA). A recent review by Asif Khan of the University of South Carolina (Columbia, SC) reports UV LEDs from 210 to 400 nm.6 Quantum efficiency at the shorter wavelengths is limited, but LEDs don’t require the high drive currents that make materials problems acute in UV lasers. Johnson and Kneissl are among researchers who have turned to UV LEDs as a more tractable problem.
Optical pumping also can produce impressively short wavelengths from nitride semiconductors, including 214 nm stimulated emission from bulk AlN epitaxial layers and 240 nm lasing from multiple GaAlN quantum wells.7, 8 But optical pumping is more useful in research than in practical devices.
Applications and outlook
It’s hard to predict how deep semiconductor diode lasers can go into the ultraviolet and how long it will take to get there. Advances on that frontier of laser technology don’t follow a neat and predictable optical counterpart of Moore’s Law. Ultraviolet laser progress has been bogged down in material problems for years; it’s too early to tell if the indium-free 342 nm laser opens the door to a practical solution. Even if diode lasers can be pushed deeper into the ultraviolet, their reliability and lifetimes are big question marks.
The biggest question mark may be whether any major applications will require diode lasers beyond 350 nm. Ultraviolet LEDs are coming on strong, and are attractive for applications that require illumination rather than a coherent beam, such as water sterilization and germicidal lamps. Light-emitting diodes also might suffice for many types of ultraviolet sensing. The market for high-definition video players pushed development of blue diode lasers, but it is unclear what will follow today’s Blu-Ray disks. Some observers expect the Internet to replace disks for video distribution, and moving far enough into the ultraviolet to multiply storage capacity would require costly new beam-focusing and readout optics as well as new lasers. In short, it’s no slam-dunk.
REFERENCES
- K. Watanabe, T. Taniguchi and H. Kanda, Nature Materials 3, 404 (2004).
- U. Schwartz, Nature Photonics 2, 521 (Sept. 2008).
- H. Yoshida et al, Nature Photonics 2, 551 (Sept. 2008).
- J. Edmond et al, J. Crystal Growth 272, 242 (2004).
- E. S. P. Leong and Siu Fung Yu, Advanced Materials 18, 1685 ( 2006).
- A. Khan, K. Balakrishnan, and T. Katona, Nature Photonics 2, 77 (Feb. 2008).
- M. Shatalov et al, Japanese J. Appl. Phys. 45, L1286 (2006).
- T. Takano, Y. Narita, A. Horiuchi, and H. Kawanishi, Appl. Phys. Lett. 84, 3567 (2004).
Tell us what you think about this article. Send an e-mail to [email protected].
About the Author
Jeff Hecht
Contributing Editor
Jeff Hecht is a regular contributing editor to Laser Focus World and has been covering the laser industry for 35 years. A prolific book author, Jeff's published works include “Understanding Fiber Optics,” “Understanding Lasers,” “The Laser Guidebook,” and “Beam Weapons: The Next Arms Race.” He also has written books on the histories of lasers and fiber optics, including “City of Light: The Story of Fiber Optics,” and “Beam: The Race to Make the Laser.” Find out more at jeffhecht.com.