Photonic Frontiers: Disk Lasers: Higher powers and shorter pulses from thin-disk lasers
Invented two decades ago by Adolph Giesen, now at the German Aerospace Center (Stuttgart, Germany), the thin-disk laser radically reshaped solid-state geometry from the traditional long, thin rod into a flat disk. Slab lasers had shown that increasing the ratio of surface area to volume could improve heat dissipation to allow higher-power operation. Thin disks are a logical extreme of the slab design: attaching a disk 0.1 to 1 mm thick to a heat sink to quickly dissipate residual pump energy. The other side of the centimeter-scale disk is pumped with diode laser beams spread across the surface.
Now scaled to multi-kilowatt powers, thin-disk lasers compete with fiber lasers for industrial applications. Both have brought impressive efficiency to solid-state lasers, but their properties differ in important ways. Fiber lasers can generate higher continuous-wave (CW) powers, but thin disks can produce higher peak powers. That makes them attractive for a wide range of applications, from precision cutting of high-strength glass for smartphones to pumping ultrashort pulse lasers in research applications.
Basics
Figure 1 shows two generic designs for diode pumping of thin disks, generally 0.1 to 1 mm thick and 5 to 15 mm in diameter. In the single-disk laser, pump diodes or arrays illuminate the surface of the disk from the side, exciting the laser species-usually ytterbium in a crystal-and then being reflected from the rear surface to pass back through the disk, exciting more atoms. A reflective layer behind the disk forms a resonant cavity with the output mirror, generating the laser beam, which in this simple example is emitted perpendicular to the disk surface. Two or more disks also can be put in series optically in a folded cavity, as shown in the dual-disk W-cavity laser, increasing gain inside the cavity and output power.In practice, the optics are more complex. More complex resonator designs are needed for high beam quality. Because the disk is so thin, the pump light may be repeatedly redirected onto the disk so it makes multiple passes through the disk, increasing beam absorption and optical-to-optical conversion efficiency. The pump intensity is high, but lower than that in a fiber laser because it is spread across the surface of the disk, not focused into the outer core of a dual-clad fiber, which typically measures 125 to a few hundred micrometers. Heat dissipation through the thin disk is quite efficient, and because the thermal gradient is linear through the thickness of the disk, thermal lensing is small.
Output mirrors typically reflect most light back into the cavity to keep gain highly saturated, with only a few percent transmitted in the beam, allowing the use of intracavity harmonic generators. This optical isolation is desirable for applications where light reflected from the workpiece otherwise might be coupled back into the laser.
High-power CW operation
Thin-disk lasers "have some very nice properties for continuous-wave operation," says Alexander Killi of Trumpf Laser (Schramberg, Germany), which makes a family of thin-disk lasers rated for CW output from 1 to 16 kW. Single-disk systems emit up to 6 kW, with four-disk lasers reaching 16 kW. Output power scales with the cross-section of the beam on the disk. Optical-to-optical conversion efficiency can exceed 50%. "The [diode] beam quality needed to pump a centimeter-scale disk laser is quite low," compared to the high brightness diodes needed to pump a fiber laser with its much smaller core. Lower-brightness pumping is an advantage because the pumps are less expensive than in high-brightness diodes.
Higher powers have been demonstrated. At CLEO 2013, Giesen said single thin disks had generated more than 10 kW with optical conversion above 50%. In August 2013, Boeing Directed Energy Systems (Albuquerque, NM) demonstrated a 30 kW CW thin-disk laser powered by 90 kW of electricity for DARPA's Robust Electric Laser Initiative. At CLEO, Giesen predicted that single-disk lasers could exceed 100 kW, and that combining a multidisk oscillator with amplifiers containing additional disks could produce megawatt-class output with good beam quality.1
High powers have come with some loss of beam quality; Trumpf's 16 kW laser has beam quality of 8 mm mrad, corresponding to a m2 <24. But recent experiments have demonstrated near-fundamental-mode output in the 1 kW power range.
One innovation has been shifting pumping from the usual 940 nm ytterbium absorption band to the 969 nm absorption line of the upper laser level, called "zero-phonon" pumping. Birgit Weichelt and colleagues at the University of Stuttgart found that 969 nm pumping reduced heat generation by 32%, reducing diffraction and increasing optical conversion efficiency to 58.5%, allowing them to generate 742 W output with m2 close to 1.5.2 Optical efficiency reached 72% in multimode operation, but m2 was about 15. Later, a team at DSO National Laboratories (Singapore) reached 1.1 kW with beam quality m2 <1.4 using a simple resonator in an evacuated chamber.3
Now, Trumpf has taken another big step to 4 kW with m2 = 1.38 from a single thin disk on a water-cooled heat sink. The laser disk has a concave surface, and one of the cavity mirrors has a convex curvature, as shown in Figure 2. The resonator cavity was in clean air, and the pump cavity directed the pump light to make 44 passes through the laser disk.4 Many of the optical and mechanical components were the same as used in Trumpf's commercial lasers, but pumping was with six 1.2 kW wavelength-stabilized pump diodes emitting at 969 nm.Pulsed operation and pumping
The disk laser is "especially well suited for pulsed operation," says Killi. "Its big advantage is that it has virtually no nonlinearity" because light in the cavity passes through only very little of the solid laser medium. The glass in fiber lasers is not highly nonlinear, but the light is concentrated in a small core, producing high densities along the length of the fiber. That causes strong Brillouin scattering and other nonlinearities that limit output of a continuous singlemode fiber laser to about 10 kW. That limit applies to peak power as well as to continuous output.
Thin disks disperse light over a larger area of the disk, and the beam propagates mostly through air in the laser cavity, so nonlinear effects are insignificant in comparison. "In a disk laser, you are kind of free, and can have almost arbitrary peak power without nonlinearities," says Killi. Repetitive, short, high-power pulses are important for many materials-working applications. Sven Schad of Trumpf says drilling of holes in automotive fuel-injection nozzles with 10 ps disk lasers allowed Robert Bosch GmbH (Stuttgart, Germany) to increase engine fuel efficiency by 20%—a feat that won the German Future Prize.
The appeal of picosecond lasers is cold ablation, which blasts the surface layer without heating or damaging the bulk material. Other important examples are cutting semiconductors or the hardened glasses used in smartphones. These are "very nice applications, which can cut arbitrary materials," says Schad.
Modelocking can generate picosecond pulses, but the challenge has been to generate the high pulse energies needed for ablation. The development of active multipass cells, such as the one shown in Figure 3, has proved a crucial advance. The first stage, on the left, uses a semiconductor saturable absorber mirror (SESAM) to start and stabilize modelocking. The second, on the left, is the active multipass cell, which folds the beam to generate a cavity length of 42.7 m, corresponding to a repetition rate of 3.51 MHz. At this rate, generating pulse energies of 40 μJ can produce average powers of 145 W.5 Commercial picosecond disk lasers generate average powers to 100 W at the 1030 nm fundamental wavelength, or 60 W at 515 nm in the green.Ursula Keller's group at the Swiss Federal Institute of Technology (Zurich, Switzerland) has cranked up the power further by operating a modelocked thin-disk laser in a vacuum to reduce the nonlinear effects of air. At CLEO 2013, the group reported record average output of 275 W with 16.9 μJ pulses at a 16.3 MHz pulse rate; pulse duration was 583 fs and peak power 25.6 MW.6
Outlook
More is coming. Picosecond disk lasers are also being developed as pumps for optical parametric chirped-pulse amplifiers generating few-cycle pulses. Catherine Teisset and colleagues at Trumpf Scientific Lasers (Unterföhring-München, Germany) have reached average power of 300 W from a disk laser emitting 30 mJ, 1.6 ps pulses at 10 kHz. They said this was the highest average power yet demonstrated from a regenerative amplifier.7 That technology could be used to drive the Extreme Light Infrastructure to produce attosecond extreme-ultraviolet pulses.
Developers also have demonstrated thin-disk lasers made from other materials. Several ytterbium hosts are being explored. Giesen's group has pumped chromium-doped zinc-selenide thin disks with both antimonide diode lasers at 1.85 μm and 1.908 μm thulium-fiber lasers to produce over 1 W at 2.35 μm.8 They have also tested holmium-YAG thin-disk lasers. It will be interesting to see what other materials make good thin-disk lasers.
REFERENCES
1. A. Giesen, "Scaling thin disk lasers to high power and energy," CLEO 2013, CTu1O.1 (2013).
2. B. Weichelt et al., Opt. Lett., 37, 3045 (Aug. 1, 2012).
3. Y. H. Peng et al., Opt. Lett., 38, 10, 1709 (May 15, 2013).
4. T. Gottwald et al., "Recent developments in high power thin disk lasers at TRUMPF Laser," Proc. SPIE, 88980P (Oct. 15, 2013); doi:10.1117/12.2028656.
5. D. Sutter et al., "Ultrafast disk lasers and amplifiers," Proc. SPIE, 82350X (Feb. 9, 2012); doi:10.1117/12.906905.
6. C. Saraceno et al., "Pushing the limits of high-power modelocked thin disk lasers by operating in a vacuum environment," CLEO 2013, CTh1H.4 (2013).
7. C. Teisset et al., "300 W picosecond thin-disk regenerative amplifier at 10 kHz repetition rate," Advanced Solid-State Lasers Congress, JTh5A.1 (2013).
8. G. Renz et al., "Cr:ZnSe bulk and Cr:ZnSe thin disk CW lasers," Lasers, Sources and Related Photonic Devices Technical Digest, AT4A.3 (2012).
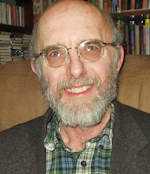
Jeff Hecht | Contributing Editor
Jeff Hecht is a regular contributing editor to Laser Focus World and has been covering the laser industry for 35 years. A prolific book author, Jeff's published works include “Understanding Fiber Optics,” “Understanding Lasers,” “The Laser Guidebook,” and “Beam Weapons: The Next Arms Race.” He also has written books on the histories of lasers and fiber optics, including “City of Light: The Story of Fiber Optics,” and “Beam: The Race to Make the Laser.” Find out more at jeffhecht.com.