Fiber Lasers: High-power, single-mode fiber lasers advance
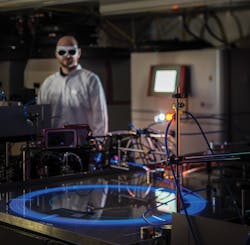
If there is one obvious trend in laser technology, then it is the rise of fiber lasers. Fiber lasers took over market shares from high-power CO2 lasers, as well as from bulk solid-state lasers in high-power cutting and welding. Major fiber laser manufacturers are currently addressing a number of new applications to conquer even more markets.
Among those high-power lasers, the single-mode systems offer features that make them desirable: They possess the highest brightness, and they can be focused down to a few microns and to the highest intensities. They also exhibit the largest depth of focus, which makes them best suited for remote processing. Still, they are difficult to manufacture and only market leader IPG Photonics (Oxford, MA) offers a 10 kW system with single-mode power. Unfortunately, there are no details available on their beam characteristics, particularly any possible multimode components that may exist along with the single-mode beam.
Funded by the German government and in collaboration with TRUMPF (Ditzingen, Germany), Active Fiber Systems, Jenoptik, and the Leibniz Institute of Photonic Technology, a team of scientists from Friedrich Schiller University and the Fraunhofer Institute for Applied Optics and Precision Engineering (all in Jena, Germany) analyzed the challenges for upscaling such lasers and then developed new fibers to overcome the limitations. The team successfully completed a series of tests showing 4.3 kW single-mode output, in which the fiber laser output was limited only by the input pump power.
Deterring effects for single-mode fiber laser upscaling
What are the challenges for such a single-mode high-power fiber laser? Those can be grouped into three fields: a) improved pumping, b) designing an active fiber with low optical losses working in single-mode operation only, and c) measuring the resulting radiation correctly. In this article, we will assume that a) is solved with high-brightness laser diodes and appropriate incoupling techniques, and will focus on the two other fields.
Within the design of an active fiber for high-power single-mode operation, there are two general sets of parameters to optimize: doping and geometry. All parameters have to be determined for minimal losses, single-mode operation, and, finally, high-power amplification. A perfect fiber amplifier would deliver a high conversion rate of more than 90%, perfect beam quality, and an output power limited only by the available pump power.
However, upscaling a single-mode system to higher powers could lead to a higher power density inside the active core, an increased thermal load, and a number of nonlinear optical effects such as stimulated Raman scattering (SRS) and stimulated Brillouin scattering (SBS).
Most remarkable is an effect that is typical for ytterbium-doped silica fibers, and is well known from the early days of fiber lasers when the fiber material was not as pure as it is today—photo-darkening. In this process, defect centers or color centers are formed in the material as a result of laser-material interaction. This effect is parasitic: it converts pump photons into heat, which results in less amplification and increased thermal load.
Depending on the size of the active core, several transverse modes can be excited and amplified. For a given index step between core and cladding, the smaller the active core cross-section, the smaller is the number of such modes. However, smaller diameter also means higher power density. A few tricks such as bending the fiber add losses for higher modes.
Still, for larger core diameters and under thermal load, other modes can arise. Those modes are subject to interactions during amplification—without optimal propagation conditions, the output profile might become spatially or temporally unstable.
Transverse mode instabilities
Ytterbium (Yb)-doped fibers are a typical workhorse medium for high-power single-mode fiber lasers. But beyond a certain threshold, they show an entirely new effect—so-called transverse mode instabilities (TMIs). At a specific power level, higher modes or even cladding modes appear suddenly, the energy is transferred dynamically between these modes, and the beam quality is reduced. The beam starts fluctuating at the output.
Since TMI has been discovered, it has been observed in various fiber designs from step-index fibers to photonic-crystal fibers. Only its threshold varies with geometry and doping, but a rough estimation says that this effect shows up beyond 1 kW output power. In the meantime, it was found that the effect is coupled to thermal effects inside the fiber with a strong relation to photo-darkening effects. Furthermore, the susceptibility of fiber lasers to TMI seems to be affected by the modal content of the core.
The geometry of a step-index fiber gives rise to a number of parameters for optimization. The core diameter, the size of the pump cladding, and the index of refraction difference between core and pump cladding can all be tuned. This tuning depends on the dopant concentration—that is, the concentration of Yb-ions can be used to control the absorption length of the pump radiation in the active fiber. Other dopants can be added to reduce thermal effects and control the refractive index step.
But there are some opposing requirements. To reduce nonlinear effects, the fiber should be shorter. However, to reduce thermal load, the fiber should be longer. Photo-darkening scales with the square of the dopant concentration, so longer fibers with lower doping would also be better.
First suggestions for these parameters can be found in simulations. Some parameters such as thermal behavior can be simulated but are difficult to predict, especially as photo-darkening is low on purpose and cannot be measured by accelerated tests. Therefore, direct measurements of the thermal behavior in fibers can be helpful for the planning of experiments.
For a typical active fiber, Fig. 1 shows a comparison of measured thermal load extracted from simultaneous distributed temperature measurements inside the fiber amplifier and the simulated heat load. To accurately predict the longitudinal temperature profile, an additional loss of only 2 dB/km was assumed, showing a very low loss.
Another important parameter for fiber design is the cut-off wavelength, which is the longest wavelength that allows for more modes inside the active core. Higher order modes beyond this wavelength are not supported.
Beyond the properties of the fiber itself, there are several means to influence the amplification process and the loss mechanisms, such as fiber bending or the temporal and spectral properties of the seed beam.
Testing new fibers toward kilowatt power
After extensive simulations, two types of Yb-doped fibers were produced and examined in a recent experiment.1 Fiber 1 had a core diameter of 30 μm and co-doping of phosphorus and aluminum. Fiber 2 had a smaller diameter of 23 μm and was co-doped to a lower degree, but contained more ytterbium to achieve a slightly higher index profile compared to Fiber 1 (see Table 1).
The calculated cut-off wavelength is located near 1275 and 1100 nm for Fiber 1 and Fiber 2, respectively. This is much closer to single-mode than for typical fiber with a core diameter of 20 μm and a numerical aperture (NA) of 0.06, which has a cut-off wavelength of ~1450 nm. The amplified laser wavelength was centered at 1067 nm.
Both fibers have been tested in a high-power pumping scheme (see Fig. 2). The pump diode laser and the seed signal were free-space coupled into the fiber, which was prepared with spliced end-caps and water-flushed connectors in a bath of still water for cooling. The seed was a phase-modulated external cavity diode laser (ECDL), which was pre-amplified to achieve a seed power of 10 W at 1067 nm and a spectral linewidth of 180 pm.Within the test of Fiber 1, sudden fluctuations were seen on the millisecond scale at a threshold of 2.8 kW, which can be attributed to TMI. Fiber 2, with a length of 30 m and the same seed linewidth, was pumped up to an output power of 3.5 kW—limited by SBS, but not by TMI.
In a third experiment, the seed laser spectrum was modified to increase the SBS threshold of the fiber by spectral broadening to a degree higher than the previous experiment. For this purpose, a second diode laser with a 300-pm-shifted central wavelength was combined with the first one. This interference leads to a temporal beating that lets the bandwidth increase with power because of self-phase modulation. In the same main amplifier as before, very similar output power values and slope efficiencies of 90% were obtained, but could be scaled to 4.3 kW without any indication of TMI (see Table 2).
Measurement challenges
Measuring all aspects of a high-power fiber laser is a major effort and requires specific equipment for several different tasks. For a full characterization of the fiber, dopant concentration, refractive index profiles, and fiber core attenuation were determined. For example, the measurement of the core loss for different bending diameters can be valuable for correlation with the TMI threshold.Spectral and temporal measurements can be executed with conventional technology. They allow the detection of effects such as the onset of SBS (a temporal signature different to TMI) or SRS (a spectral signature). Care should be taken to measure with a high dynamic range to see early growth of parasitic spectral features, like amplified spontaneous emission or SRS. Such a high dynamic spectrum is shown in Fig. 4b and proves that SRS is not detectable.
Beam quality measurements are the most difficult part of fiber laser characterization and deserve a separate discussion. Briefly, attenuation without introducing thermal effects is the key, and could be done with Fresnel reflections or low-loss transmission optics.
In the experiments presented here, the attenuation was done using wedge plates and pulsed pumping at a timescale longer than that for onset of TMI. At an output power of 4.3 kW, an m2 of 1.27 in the x-direction and of 1.21 in the y-direction was measured.
Applications in ultrafast science
After about a decade of stagnation in upscaling high-power single-mode fiber lasers, it now seems feasible to develop a new generation of kilowatt-class fiber lasers with excellent beam quality. Output powers of 4.3 kW have been shown, limited only by the pump power. Major limitations for further upscaling are identified and ways to overcome these limitations have been identified.
It should be noted that it was the careful investigation of all known effects and a subsequent parameter optimization that led to the advances in fiber design and, finally, to new records in output power. A further upscaling and adaption of the fiber for other applications seem feasible and will be targeted next.
This opens up a number of interesting perspectives. On the one hand, transferring the results into industrial products is desired by the project partners, but will require additional major development efforts. On the other hand, this technology is highly relevant for the upscaling of other fiber laser systems, such as femtosecond fiber amplifiers.
In fiber amplification of ultrafast laser pulses, almost 1 kW has already been achieved from a single fiber2—upscaling towards 5 kW now seams feasible by combining techniques.3 While such systems are in development for research centers such as ELI (Prague, Czech Republic), it remains a major challenge for industrial systems to develop reliable means for beam transport.
Both the upscaling of single-mode fiber lasers and of femtosecond fiber amplifiers will require major additional research effort. This effort will be supported by an entirely new building beside Fraunhofer IOF. This new fiber technology center building was completed in 2016, and will house special laboratories for the manufacturing and characterization of active and passive fibers, as well as nanostructured optical fibers. A separate drawing tower for manufacturing special fibers will also be installed.
REFERENCES
1. F. Beier et al., "Single-mode 4.3 kW output power from a directly diode-pumped Yb-doped fiber amplifier," to be published in Opt. Express.
2. T. Eidam et al., Opt. Lett., 35, 94–96 (2010).
3. M. Müller et al., Opt. Lett., 41, 3439–3442 (2016).
About the Author
Thomas Schreiber
Group Leader, Fiber Laser Research at Fraunhofer IOF
Thomas Schreiber is group leader for fiber laser research at the Fraunhofer Institute for Applied Optics and Precision Engineering (Fraunhofer IOF; Jena, Germany).
Andreas Tünnermann
Andreas Tünnermann is director of the Fraunhofer Institute for Applied Optics and Precision Engineering (Fraunhofer IOF), as well as a professor in physics and director of the Institute of Applied Physics at Friedrich Schiller University (both in Jena, Germany).
Andreas Thoss
Contributing Editor, Germany
Andreas Thoss is the Managing Director of THOSS Media (Berlin) and has many years of experience in photonics-related research, publishing, marketing, and public relations. He worked with John Wiley & Sons until 2010, when he founded THOSS Media. In 2012, he founded the scientific journal Advanced Optical Technologies. His university research focused on ultrashort and ultra-intense laser pulses, and he holds several patents.