Lasers for the Biosciences: Novel ultraviolet 320 nm laser source enhances flow cytometry
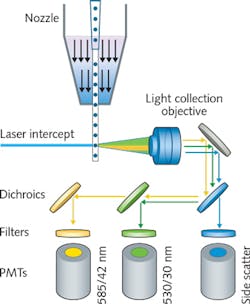
Flow cytometry is a fundamental technique in the biomedical sciences in which molecular fluorescent tags attached to cells are excited using lasers, and detected using photomultiplier tubes (PMTs) and other light-sensing technologies (see Fig. 1). Cells are introduced into the laser beam in a hydrodynamically focused liquid stream.
Some flow cytometers only analyze fluorescent tags attached to the surface or interior of cells. In fluorescence-activated cell sorters (FACS), the liquid stream is broken up into droplets and the droplets electrostatically charged and diverted into collection tubes. Cell sorting allows the separation and collection of cells based on fluorescent tagging, allowing the collected cells to be used for functional studies and proteomic and genomic analysis.
Flow cytometry has been a critical tool in expanding our understanding of the immune system, cancer biology, and infectious disease. The fluorescent probes used to label cells for this technique can identify individual cell types in complex mixtures, as well as the physical and physiological characteristics of single cells.
Background on lasers for flow cytometry
Flow cytometry is absolutely dependent on laser technology to excite these fluorescent tags.1 The earliest flow cytometers employed single lasers (at the time, usually large-water-cooled ion lasers) to excite at most one or two fluorescent probes, including common fluorochromes like fluorescein and rhodamine. Modern flow cytometers now use solid-state lasers with wavelengths spanning the entire visible spectrum from the ultraviolet (~355 nm) to long red (~700 nm).
Advanced cytometers can be equipped with as many as 10 distinct single-wavelength lasers, allowing excitation of a huge variety of fluorescent probes with differing excitation/emission characteristics. While early instruments could only excite and detect one or two probes, simultaneous detection of 30 or more fluorescent probes is now possible on advanced instrumentation. This high-dimensional analysis has expanded our knowledge of the immune system to unprecedented levels.
Modern flow cytometers use many laser wavelengths and corresponding fluorescent probes for simultaneous analysis of 20 or more cell characteristics. Advanced instruments will usually be equipped with a cyan laser (488 nm), a red laser diode (~640 nm), and a green, green-yellow, or yellow diode-pumped solid-state (DPSS) laser (532, 552, or 561 nm). These lasers all excite a variety of fluorochromes (see Fig. 2).Violet laser diodes are important excitation sources with the development of the Brilliant Violet (BV) polymer dyes by BD Sirigen.2 Seven BV dyes (BV421, BV480, BV570, BV605, BV650, and BV787) are available that are spectrally compatible, dramatically increasing the number of simultaneous cell markers that can be analyzed. Ultraviolet (UV) lasers have achieved new importance in flow cytometry with the recent development of six Brilliant Ultraviolet dyes (BUV395, VUV496, BUV563, BUV661, BUV737, and BUV805), taking the total number of simultaneous fluorescent analyses to nearly 30. UV lasers are now a critical component for advanced flow-cytometry instrumentation.
Modern flow cytometers primarily rely on frequency-tripled neodymium-doped vanadate (Nd:YVO4) 355 nm solid-state lasers to excite the BUV and other UV-excited dyes. These lasers are a considerable improvement over the argon-ion and krypton-ion lasers previously needed to provide UV excitation, as they are smaller and much easier to maintain. However, they remain expensive and are the single most costly component on higher-end instrumentation.
Less-expensive near-UV laser diodes (375 nm) can be substituted for 355 nm sources for many UV-excited dyes, including the BUVs.3 However, their wavelength is very close to the emission range of BUV395, the shortest of the series, making it difficult to detect. A less-expensive solid-state UV-laser option is therefore needed to lower the cost of high-dimensional flow cytometry.
Compact 320 nm laser module
This situation has changed with the recent development of a small solid-state 320 nm laser module with continuous-wave (CW) emission in a small air-cooled unit, developed by LASOS (Jena, Germany). Flow cytometers have historically been equipped with helium-cadmium (HeCd) lasers, taking advantage of their 325 nm laser line to excite UV dyes. However, HeCd lasers are large, not very powerful, and develop noise issues as they age. A 320 nm source would be very close in wavelength to this legacy wavelength, and would be predicted based on excitation spectra to excite the BUV dyes reasonably well.
To test whether this laser wavelength and source were applicable for flow cytometry, a module was installed on a BD LSR II flow cytometer in place of the conventional 355 nm UV laser source (see Fig. 3a).4 The laser was then aligned in to the liquid stream used to inject cells into the laser path. The optics of the instrument originally configured for the 355 nm laser source remained efficient down to 320 nm, with broadband UV mirrors used to steer the laser to the sample stream and fused silica lenses to focus the beam.Flow cytometry uses fluorochrome- tagged polymer microspheres as simulated samples to determine if instrument operation is optimal, and to test new laser sources. A mixture of dim and bright UV-excited microspheres (InSpeck Blue microspheres; Thermo Fisher, Eugene, OR) were also analyzed-resolution of the dimmest populations is an indicator of good excitation and detection. These microspheres, including the dimmest populations, were easily resolvable with this laser source (see Fig. 3b). Sensitivity was similar to a 355 nm laser at an equivalent power level. Mouse spleen cells were then tagged for a cell-surface marker using an antibody coupled to BUV563 and BUV661, two of the BUV dyes. The 320 nm module excited these samples nearly as well as the 355 nm source (see Figs. 3c and 3d).
The LASOS 320 nm laser source therefore proved to be a good replacement for 355 nm excitation in flow cytometry. These modules are smaller and less costly than most 355 nm sources, making them a cost-effective replacement for high-dimensional flow cytometry. Small solid-state laser sources both in this range and throughout the visible spectrum have revolutionized flow cytometry, decreasing the size, cost, and maintenance needs of modern instrumentation. This is an encouraging trend in technology development and will continue to contribute to better biomedical analysis instrumentation.
REFERENCES
1. W. G. Telford, Methods Cell Biol., 102, 375–410 (2011); doi:10.1016/b978-0-12-374912-3.00015-8.
2. P. K. Chattopadhyay et al., Cytometry A, 81, 456–466 (2012).
3. W. G. Telford, Cytometry A, 87, 1127–1137 (2015).
4. W. G. Telford, L. Strickland, and M. Koschorreck, Cytometry A, 91, 314–325 (2017).
About the Author
Marco Koschorreck
Head of R&D, Jenoptik Laser
Marco Koschorreck is Head of R&D at Jenoptik Laser (Jena, Germany).
Lynn Strickland
Business Development and Customer Support, LASOS North America
Lynn Strickland is in Business Development and Customer Support at LASOS North America (San Diego, CA).
William Telford
Senior Associate Scientist, Experimental Transplantation and Immunology Branch
William Telford, Ph.D. has more than 20 years experience in flow cytometry. He is extensively involved in both national and international cytometry education programs, including the National Flow Cytometry Resource Flow Cytometry Workshops (as a sustaining faculty member) and the International Society for Advancement of Cytometry, where he has taught numerous courses, tutorials and workshops over the last 10 years. He maintains a small independent research and development program in the Facility aimed at both hardware and wetware development, particularly in the area of novel laser technology, and has published extensively in this area.