Advanced wakefield laser accelerator hits energy milestone
A compact particle accelerator less than 20 meters long, which produces an electron beam with an energy of 10 billion electron volts (10 GeV), was recently demonstrated by an international team of researchers led by Bjorn “Manuel” Hegelich, an associate professor of physics at The University of Texas at Austin (see video).
Only two other accelerators within the U.S. can reach such high electron energies, and they’re both about 3 kilometers long. In a Texas-sized breakthrough, the team’s laser accelerator can achieve these energies in 10 centimeters instead (the size of the chamber that produces the beam).
Hegelich is fascinated by the fact that lasers are so powerful they can turn an electron relativistic instantaneously. In other words: any electron exposed to the laser will immediately acquire its rest mass energy according to the most famous physics equation of them all: E=mc2—within one blazingly fast femtosecond.
“A femtosecond compared to one second is like one second compared to the age of the universe, so it’s instantaneous,” Hegelich says. “If you increase the laser intensity even further, you start to get into quantum effects and there are ideas of how we might use it to test quantum gravity. From a fundamental physics side, it’s a very fascinating regime to explore.”
From a practical standpoint, Hegelich is driven by the potential to create a real impact. “The first computers were building-sized machines and could do amazing stuff in their time,” Hegelich says. “But computers started to have a really broad impact once we figured out how to make them small enough that everyone could have them. Accelerators and x-ray sources driven by accelerators have been our most powerful research tools for decades. But if we democratize access, make them small and compact enough that they become ubiquitous, we can realize their full potential.”
Wakefield laser accelerators
The concept of wakefield laser accelerators (see Fig. 1) was first described back in 1979: an extremely powerful laser strikes helium gas, heats it into a plasma, and creates waves that kick electrons from the gas out into a high-energy electron beam.
“In our basic setup, we have a gas cell or jet with helium or hydrogen or some mixture,” says Hegelich. “An ultra-intense laser travels through that gas, ionizes it, and turns it into a plasma. The laser pulse drags a plasma wave behind it like a boat going across a lake drags a wake (see Fig. 2). Electrons can surf that wake, get dragged along and gain energy, and get accelerated.”
These laser fields are super strong, so the wave is also very strong, and the electrons get accelerated hard. “In our case, we then seed this setup with metallic (aluminum) nanoparticles. When the laser ionizes them, many electrons are injected into the wave, so we can get more charge. And because of that excess charge we can also keep the accelerations going longer—reaching even higher energies.”
A big surprise was in store for the team when (based on previous results at a smaller laser) they expected a smaller energy spread around 1.5x higher energy and less charge when using the nanoparticles—but instead got a stunning 5x higher charge.
“Once we started shooting at our large Texas Petawatt Laser, we saw right away that things were more than just a little different than before—they were very different,” says Hegelich. “For 10 years, we’d tried to increase the electron energy without much success; we were stuck around 2 GeV. As soon as we used the nanoparticles, the energies were much higher and we got many more electrons.”
It’s just the beginning—now they’ve shown the principle works. Next up: precision control. “A large part involves laser engineering to develop lasers with much higher shot rates, plasma engineering to achieve precision, and software engineering to achieve better feedback and control,” says Hegelich. “This will make our result of reaching 10 GeV truly applicable and enable applications in semiconductor metrology and production, space physics, and cancer therapy and diagnostics.”
Texas Petawatt Laser desperately needs funding
The main challenge for the team’s demonstration was that the Texas Petawatt Laser fires only about four or five shots per day—and they don’t necessarily all work the way they should.
“Laser parameters like energy, pulse duration, or focal spot size can fluctuate quite a bit because parts of the system are quite old,” explains Hegelich. “Other parts are new and upgraded, but the main amplifiers (see Fig. 3) come from Lawrence Livermore National Laboratory’s old Nova laser that was decommissioned in 1999, so we typically get only one or two good shots per day and overall statistics are quite poor. We wanted to ensure the results are repeatable, so we executed three experimental campaigns during two years to get the data (see Fig. 4).”
The team could rebuild the Texas Petawatt Laser quite differently or even upgrade the current laser to fire once a minute rather than once every few hours. Sadly, so far, this upgrade hasn’t been funded. Quite the opposite is happening—funding for the laser (even in its current state) will run out in 2024. “Unless we find a new source of funding, we might need to shut the facility down,” Hegelich adds.
Wide range of applications ahead
In the near term, at lower energies, the team’s technology may find applications in semiconductor metrology for three-dimensional (3D) imaging of new 3D chip designs. “It’ll also find applications in radiation testing of space-bound electronics,” says Hegelich. “Health is another key sector—there’s demand for our laser technology in therapeutics where we’ll see very-high-energy electron therapy for cancers and also in diagnostics where it enables new methods like x-ray-induced acoustic computed tomography.”
One huge potential application is a laser-driven x-ray free-electron laser (XFEL) like the Linac Coherent Light Source at Stanford University or the University of Hamburg’s EuroXFEL, which needs a >10-GeV electron beam to drive it.
Merely a handful of these machines currently exist worldwide, which means limited access and limited available time to use them. “Hard XFELs are the ultimate x-ray microscopes, achieving molecular resolution in both space and time, so they’ll become the cutting-edge research tools for everything from materials science to biology, chemistry, and more,” Hegelich says. “They’ll enable research for new batteries, solar cells, designer pharmaceuticals, vaccines, drugs, and hundreds of other industries.”
The team’s technology can shrink XFELs “from campus to lab size, so you could have one at every large university or even companies,” he adds. “This will democratize access, making beam time accessible to more researchers and industry than previously possible.”
Scaling to lower laser pulse energy
The researchers are currently working to scale down to lower laser pulse energy—while retaining the advantages they’ve observed—to reach higher electron energies with a given laser or reach a target electron energy with a smaller laser.
“We’re going to work on the precision control of the mechanism at a higher shot rate—100 shots per second and more—at our applications center in Carlsbad, CA, with TAU Systems Inc.,” says Hegelich, who founded the company. “We’ll explore the scaling of multiple acceleration stages, starting with two stages, then more.”
Hegelich and his team are also working on developing a multi-kilohertz drive laser.
FURTHER READING
C. Aniculaesei et al., Matter Radiat. Extremes, 9, 014001 (2024); https://doi.org/10.1063/5.0161687.
Related Content
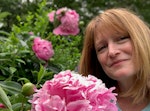
Sally Cole Johnson | Editor in Chief
Sally Cole Johnson, Laser Focus World’s editor in chief, is a science and technology journalist who specializes in physics and semiconductors.