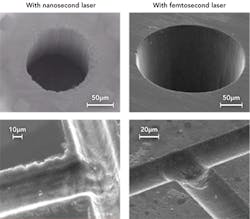
SEYDI YAVAŞ
Glass is a transparent and solid-like material with diverse areas of application in our daily lives. It is a boundless material whose applications' diversity is growing, finding more uses in combination with other materials for high-tech applications. Glass is made from easy-to-find materials such as soda ash, limestone, and sand. These materials melt at very high temperatures (around 1500°C) so that it behaves like liquid and can be poured, blown, pressed, and molded into a multitude of forms. However, at ambient temperature, it acts like a solid and becomes harder to process after cooling due to changes in mechanical properties.
Glass has unique capabilities that no other materials have. It has fascinating optical properties such as reflecting, bending, transmitting, and absorbing light, and has high bandgap for transparency over the entire visible range and beyond. Chemically, it is corrosion-resistant and inert so that it can be used as a container for most chemicals. Thermally and electrically, it is an excellent insulator. Mechanically, a glass surface is hard so that it is scratch- and abrasion-resistant, and in recent years with various methods, it even gained elasticity. However, these same properties render processing of glass challenging—for example, while glass has great tensile strength, it is brittle.
Consequently, the history of methods to treat glass is as rich as the history of its applications. Glass producing has an ancient tradition that dates back to around 3500 BC. It is assumed to have been first artificially manufactured in Mesopotamia and Egypt to be used as jewelry and afterward as jugs. Since then, processes have continuously evolved from workmanship to today's high-tech industrial methods, and numerous glass types and uses have emerged. Even though the manufacturing of glass has a long history, in the last few decades, processes made using already-produced glass have been limited due to its brittleness.
Breaking and disintegrating of glass commonly begins with a small crack. After the crack forms in some part of the body, it travels and reaches the edge and causes it to break. This brittle nature of glass makes it hard to process. On the other hand, developing technology necessitates smaller structures to be formed in different types of glasses for many application areas. There are conventional precise methods like photolithography and electron beam lithography that can also process glass, but both of these techniques are expensive and hard to operate, especially on large areas. Today, laser-based techniques offer the most precise ways of processing glass. The most straightforward approach relies on the use of single-photon absorption in wavelength ranges where glass is not highly transparent-either in the infrared (IR) or ultraviolet (UV).
However, direct absorption leads to problems, including undesirable thermal effects and formation of heat-affected zones, which can severely compromise mechanical stability by seeding micro-crack propagation. Furthermore, processing substantially below the surface, towards the creation of 3D structures, requires use of wavelengths of high transparency. While nanosecond-pulsed lasers can be used to create sub-surface structures in glass (FIGURE 1), the physical mechanisms involved also impose limitations to the finesse of the micromachining and can also cause micro-cracks.
In recent years, an exciting alternative has become practical enough for industrial usage—namely, the use of ultrafast lasers producing sub-picosecond pulses in near-infrared (NIR) wavelengths. In this approach, ultrashort pulses are focused tightly into the bulk of glass or its surface, with power densities exceeding terawatts per square centimeter that trigger exotic processes such as simultaneous absorption of multiple photons and avalanche and impact ionization, resulting in a highly localized disruption of the glass matrix with very little energy deposited (a few microjoules or less). Due to the very modest amounts of energy used per pulse, the thermal effects to the region—even immediately surrounding the focal volume—can be kept negligible. This approach is often called "cold ablation" and enables creation of extremely precise 3D structures.
Femtosecond laser micromachining of transparent materials has distinctive advantages over other micromachining techniques (FIGURE 2).Femtosecond laser micromachining arises from a phenomenon called "laser-induced optical breakdown." In this process, the optical energy of the femtosecond laser is transferred to the processed material, exciting many electrons and causing them to ionize and transfer energy to the lattice. Subsequently, a structural or phase alteration in the material occurs and causes a permanent refractive index modification or even leaving a void just at the focal spot.
Understanding why ultrafast pulses are ideal for micromachining necessitates approaching the problem from the timescale of the material processing point of view. Damage caused by a laser has long been studied, but there are important differences between damage caused by a femtosecond pulsed laser and damage caused by pulses with duration greater than picoseconds. In most of the materials, phonons that are the main reasons of thermal effects need at least one picosecond of laser exposure to be excited. For femtosecond laser pulses, exposure duration is lower than this limit.
Hence, any ions will not be excited thermally by electrons since the femtosecond pulses would end before this time period. Heat diffusion outside—even in the vicinity—of the focal area is minimal, resulting in an increase of the precision of the process. In addition, femtosecond laser material processing is highly deterministic since the seed electrons to seed the absorption process are generated through nonlinear ionization mechanism and defect electrons in the material are not required. The repeatability and confinement of the nonlinear excitation allows the use of femtosecond laser micromachining for practical purposes for which other methods are not capable.
Until recently, use of ultrashort pulses on glass was a known but not widely used method since the pulse energy need for this process is relatively high. The only lasers that were providing these pulse energy levels were solid-state lasers that are complex, difficult to operate, and expensive to purchase. Improvements in fiber laser technology over the last few years provide pulse energy levels with short pulses and high beam quality that make them an important alternative to solid-state lasers. In addition, fiber lasers are more economical, compact, reliable, and easier to operate since they are alignment-free.
The development of user-friendly, low-cost, microjoule-range ultrafast fiber lasers is now transforming this technique from a niche application to a widely adopted industrial processing tool (FIGURE 4). Applications range from product authentication to glass cutting, as well as the creation of sub-surface optical waveguides.Applications
Surface texturing and roughening using ultrashort pulses is a widely used method for metals since control over the process and precision provides amazing results, enabling applications such as biomedical implant roughening to increase cell adhesion and thin-film etching for LEDs and solar cell efficiency increments. Common methods like sand blasting and chemical etching for texturing on glass surfaces provides moderate-level precision and limited control over the pattern obtained, often causing micro-cracks that reduce the durability of the processed glass. As a result, fiber lasers find usage in surface texturing of glass, especially for applications like producing hydrophobic/hydrophilic glass surfaces. Recently, treatments using ultrashort-pulsed lasers show it is possible to get the same or even better-quality wettability conditions on glass surfaces, and they are highly promising for the future of surface treatment applications. They provide total control over the process pattern on the surface, making it possible to optimize liquid-glass interaction.
Another promising application for ultrashort-pulsed fiber lasers is the welding of glasses. The most widely used method to stick two glasses together is to glue them using chemicals. The biggest drawback of this method is that most of the chemicals used release some gases over time, causing the power of the bond between glasses to reduce due to gas in between. Another method used is to polish the glass surfaces, bringing them together and then treating with heat afterward. This method also has shortcomings, especially if two different types of glasses are used. Since they may have different heat-expansion coefficients, the bond between the glasses could become weak after heat treatment. Recently, high-repetition-rate (megahertz) and high-pulse-energy (microjoule), ultrashort-pulsed lasers have begun to be used for welding of glasses. When the ultrashort pulses are focused at the interface of two glasses to be welded together, very small volumes from each glass melt and cool down together, resulting in a very powerful bond. With correct optimization of ultrashort laser welding processes, it is possible to obtain bonds as strong as the glasses themselves (FIGURE 5).Conclusion
Femtosecond laser micromachining offers distinctive capabilities for 3D, material-independent, and subwavelength-precise processing, and also enables the fabrication of 3D structures in transparent materials such as glass with far greater simplicity than lithography. The femtosecond micromachining field is evolving at a rapid pace and this technique promises possibilities beyond the field of micromachining. It is a technology that creates new fields and markets for the laser industry and facilitates state-of-the-art applications that will affect the future of technology in many ways.
REFERENCES
1. K. Itoh, W. Watanabe, S. Nolte, and C. B. Schaffer, MRS Bulletin, 31, 620–625 (2006).
2. R. R. Gattass and E. Mazur, Nature Photon., 2, 219–225 (2008).
3. M. S. Ahsan, F. Dewanda, M. S. Lee, H. Sekita, and T. Sumiyoshi, Appl. Surf. Sci., 265, 784–789 (2013).
4. G. Della Valle, R. Osellame, and P. Laporta, J. Opt. A: Pure Appl. Opt., 11, 013001 (2008).
5. S. Richter, S. Döring, A. Tünnermann, and S. Nolte, Appl. Phys. A: Mater. Sci. Process., 103, 257–261 (2011).
6. See http://bit.ly/1nvrlCb.
7. See http://bit.ly/1yCV85F.
8. See http://bit.ly/1pOodcv.
SEYDI YAVAŞ ([email protected]) is Senior R&D Engineer – Laser Expert at FiberLAST Inc., Ankara, Turkey.