FIBER LASERS: Fiber-laser power scales coherently and spectrally
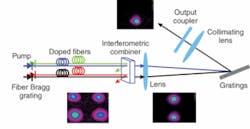
Two methods for combining the output of several fiber lasers for the purpose of power scaling are spectral addition and coherent addition. In spectral addition, fiber lasers (of slightly different wavelengths) are aligned along one direction (linearly) for incoherent combination using a linear diffraction grating. In coherent addition, beams from individual lasers are coherently added by passive or active elements; unfortunately, even though the addition can be readily performed in two dimensions, the large number of elements required limits the number of lasers that can be added. Using a new technique that simultaneously performs spectral and coherent addition of a two-dimensional (2-D) fiber-laser array, researchers at the Weizmann Institute of Science (Rehovot, Israel) can overcome the inherent upscaling limitation of each individual method.1
The combination of coherent addition in free space (to reduce the possibility of fiber damage and degrading nonlinear effects due to high powers when a single output fiber is used) and spectral addition of the now linearly oriented beams allows very large arrays of fiber lasers to be efficiently power-scaled (see figure). In the experimental setup, four end-pumped single-mode erbium-doped fibers are arranged in a 2 × 2 square array with a distance of 3.5 mm between adjacent fiber-output ends. Each 7-m-long fiber has a 10 µm core diameter and a numerical aperture of 0.19 and is attached to a fiber Bragg grating that serves as the rear mirror, while the other fiber ends are connected to a collimator coated with antireflection layers to prevent backreflection into the fiber.
The four parallel beams that emerge from these fibers are first coherently added in the horizontal direction using a passive interferometric combiner to produce two parallel beams. A lens then deflects these beams in the vertical direction such that they exactly overlap on the surface of a 1200-lines/mm blazed diffraction grating. This combined beam is then diffracted toward a common planar output coupler with 20% reflectivity; thus, the two beams have been spectrally added to maintain the high beam quality of each of the individual lasers.
The beam-quality parameter M2 for the combined output beam was measured to be 1.15, essentially identical to the beam-quality parameters for each of the four individual fiber lasers. The overall combining efficiency, after both coherent and spectral addition, was measured to be 82%, attributed mainly to losses at the blazed diffraction grating and also to small misalignments in the parallelism of the four collimated fiber-laser beams. Analysis of the spectral output of the combined beam compared to the individual spectrum of the fiber-laser beams showed that there was no interaction of the beams in the test setupthe overall output spectrum was basically the sum of the individual spectrum of each fiber laser. For lasers with a spectral separation of 1.3 nm and an overall bandwidth of 70 nm (as used in this experiment), more than 50 fiber lasers could be added using this method.
The Weizmann Institute researchers believe that the combined approach of simultaneous coherent and spectral additions of individual fiber lasers opens a route to upscaling to large arrays, overcoming the upscaling limitation of each approach. “Such upscaling could lead to a combined-lasers system with very high output powers concomitantly with good output-beam quality,” they say.
REFERENCE
1. M. Fridman et al., Optics Lett. 33(7) 648 (April 1, 2008).
About the Author
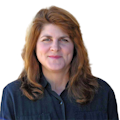
Gail Overton
Senior Editor (2004-2020)
Gail has more than 30 years of engineering, marketing, product management, and editorial experience in the photonics and optical communications industry. Before joining the staff at Laser Focus World in 2004, she held many product management and product marketing roles in the fiber-optics industry, most notably at Hughes (El Segundo, CA), GTE Labs (Waltham, MA), Corning (Corning, NY), Photon Kinetics (Beaverton, OR), and Newport Corporation (Irvine, CA). During her marketing career, Gail published articles in WDM Solutions and Sensors magazine and traveled internationally to conduct product and sales training. Gail received her BS degree in physics, with an emphasis in optics, from San Diego State University in San Diego, CA in May 1986.