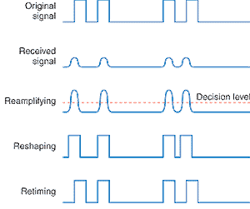
Electronic regeneration came first in fiberoptics, and optical amplification followed. Now developers are combining regeneration with optics to build optical regenerators. Optical regeneration began as a gleam in the eye of researchers who hoped to avoid the need to convert optical signals to electronic when they required regeneration. It is an unaffordable luxury at speeds to 10 Gbit/s, however, when a combination of optical amplifiers, passive dispersion compensation, and electronic regeneration at switching nodes can meet most transmission requirements.
Yet moving to 40 Gbit/s is likely to make optical regeneration into a practical necessity for long-distance transmission. The severity of chromatic dispersion increases with the square of data rate at high speeds, so a fiber that transmits 10 Gbit/s for 1000 km can transmit 40 Gbit/s only 62.5 km. The impact of polarization-mode dispersion also becomes more severe. Active dispersion management can extend the reach of 40-Gbit/s systems, but the cumulative dispersion eventually limits distance, making regeneration a necessity for long-haul 40-Gbit/s transmission.
Electronic regeneration is straightforward if expensive at 10 Gbit/s, but it is not clear whether it will prove cost-effective at 40 Gbit/s. Optical regeneration technology appears viable for data rates of 40 Gbit/s, and is the only technology available for beyond 40 Gbit/s.
The Rs of regeneration
The ideal type of regeneration is called "3R": the Rs are reamplifying, reshaping, and retiming (see Fig. 1). The number of Rs needed depends on the application.
An erbium-doped fiber amplifier is essentially a 1R regenerator that only "reamplifies" the signal. Optical amplifiers are analog devices that can add noise to the signal, and this noise builds up as a signal passes through a series of amplifiers. After too many amplifiers, the noise accumulates to cause errors in digital transmission.
The next step is 2R regeneration, which reshapes as well as reamplifies the signal. Soliton transmission provides 2R regeneration because solitons retain their pulse shape in a suitable transmission system as long as they retain the required intensity. Optical amplifiers amplify the signal, while nonlinear interactions between the optical pulses and the fiber reshape the pulse. The 2R regeneration can cope with amplitude noise but not with jitter, which causes pulses to drift away from the proper time slots.
Full 3R regeneration requires retiming in addition to reshaping and amplifying the pulse. That's a more difficult task because it requires comparing pulse timing to the clock signal, and pushing the pulses forward or backward into the time slots they should occupy.
One implicit requirement is that regenerators must be cascadable so they can be placed in series to stretch transmission distances. Noise and other signal impairments may limit the number of regenerators that can be cascaded, as with optical amplifiers, but ideally there should be enough to circle the globe.
Wavelength-division multiplexing (WDM) complicates regeneration. Most optical and electronic regenerators can process only one optical channel at a time, so WDM signals must be demultiplexed before the channels are regenerated separately. The notable exception is regeneration using synchronous modulation of soliton pulses (described later), which can simultaneously regenerate several channels if their bit periods are synchronized.
Several types of optical regenerators have been developed. One stage must have a nonlinear response to discriminate between noise below a threshold power and signal above the threshold. Amplification may be separate, or combined with the nonlinear stage, as in semiconductor optical amplifiers. Various approaches are used to extract and regenerate clock signals in 3R regenerators.
2R regenerators
The major attractions of 2R regeneration are cost and simplicity, because they do not require components to recover the signal timing.
Periodic amplification of soliton pulses can be considered a type of 2R regeneration. In soliton transmission, self-phase modulation of optical pulses in the transmission fiber offsets the pulse spreading caused by chromatic dispersion. As long as the optical power is sufficient to produce self-phase modulation, the interaction stabilizes optical pulses in the shape of solitons. This can effectively regenerate soliton pulses after an amplification stage, if they do not deviate too much from the ideal soliton shape. Soliton systems are inherently limited to return-to-zero (RZ) format.
Digital pulses also can be reshaped by a strongly nonlinear amplifier. The ideal response is generating zero output for input below a decision threshold, and high output above that threshold, performing the function of an electronic decision circuit. Mads Nielsen of the Technical University of Denmark described one example last month at OFC 2002 (Anaheim, CA). It is based on a 2 x 2 multimode interference coupler, fabricated from material that acts as a semiconductor optical amplifier, developed by Jan de Merlier at the University of Ghent. The input signal enters one of the input ports, exciting multiple guided modes in the wider middle section. The signal is amplified as it passes through the waveguide. Interference effects focus the amplified light onto a small zone on the other end. The internal gain changes the focus point by modulating the waveguide's refractive index; the lower the gain, the higher the index. The gain in turn depends on the power level, decreasing at higher powers as the material saturates. The guide is designed to switch the output between the two outputs, depending on the input power level. The response is highly nonlinear, so the output turns on as input exceeds a threshold value, reshaping the pulse (see Fig. 2). The system has been demonstrated with 10-Gbit/s signals, but can be applied at higher data rates.A saturable absorber can perform a similar decision function. Low-power noise is absorbed, but higher signal powers saturate the device so the light is transmitted, regenerating a signal with sharply defined ON and OFF levels. Using a multiple quantum-well saturable absorber, Olivier Leclerc and colleagues at Alcatel and CNRS-LPN have doubled transmission of a noisy 20-Gbit/s signal from 3400 to 6800 km in an experiment reported at the ECOC 2000 postdeadline session.
3R regeneration
Some 2R techniques can be used for 3R regeneration with addition of a circuit that recovers the clock of the input signal. Clock recovery requires dividing the input signal, and detecting part of it to identify the clock timing. From that information, a new clock signal is generated at the known input rate, with the input signal helping to set the phase.
A leading approach to 3R regeneration is built around wavelength conversion in a semiconductor optical amplifier. An input signal at one wavelength modulates either the phase or the intensity of light at another wavelength passing through the semiconductor amplifier. Several configurations have been developed. In one example, the input signal illuminates one of a pair of parallel semiconductor amplifiers arranged as a Mach-Zehnder interferometer, while both arms are amplifying a second wavelength (see Fig. 3). The input signal changes the carrier density and thus the refractive index of the illuminated amplifier, modulating the phase of the amplified light at the second wavelength. Combining the outputs of the two parallel amplifiers causes interference, which converts the phase modulation to intensity modulation, generating an output signal at the second wavelength. Nonlinear response enhances the extinction ratio, regenerating the input signal.Retiming is added by modulating the amplified wavelength with the regenerated clock signal. In this case, the wavelength converter functions as a gate controlled by the input signal, which either blocks or passes pulses at the second wavelength. The input signal can be returned to the original wavelength by using the output of the first stage to drive another wavelength converter that generates the input wavelength, and Alcatel has used that technique to cascade more than 100 optical regenerators at 40 Gbit/s.
An alternative approach to 3R regeneration is synchronous intensity and phase modulation of RZ pulses including solitons. The input pulses first pass through a narrowband filter, to stabilize amplitude fluctuations arising from jitter. Part of the input is split to generate a clock signal, which applies sinusoidal phase and amplitude to two parallel modulators arranged as arms of a Mach-Zehnder interferometer. The overall effect is to produce peak transmission at the intervals set by the clock signal, which pushes off-center pulses back to their proper time slots. This approach can be used to simultaneously regenerate multiple channels if the input signals are first demultiplexed and separate time delays applied to make the pulses on all channels synchronous with each other. Then the synchronized channels can be recombined and passed through the same synchronous modulation. Leclerc's group has demonstrated this technique for four 40-Gbit/s channels.
Encouraging prospects
Other techniques also are in development at a research level, with some aimed at specific applications, such as power-sensitive undersea systems. Nobody claims optical regeneration will be easy, but all in all it represents a potential alternative to optoelectronic regenerators for 40-Gbit/s systems when complexity, power consumption, and costs are considered, says Olivier Leclerc of Alcatel. Looking forward, Leclerc adds, all-optical regenerators "are today the only ones that can provide regeneration of optical signals at bit rates beyond 40 Gbit/s."
ACKNOWLEDGMENTS
Thanks to Olivier Leclerc of Alcatel and Mads Nielsen of the Technical University of Denmark.
About the Author
Jeff Hecht
Contributing Editor
Jeff Hecht is a regular contributing editor to Laser Focus World and has been covering the laser industry for 35 years. A prolific book author, Jeff's published works include “Understanding Fiber Optics,” “Understanding Lasers,” “The Laser Guidebook,” and “Beam Weapons: The Next Arms Race.” He also has written books on the histories of lasers and fiber optics, including “City of Light: The Story of Fiber Optics,” and “Beam: The Race to Make the Laser.” Find out more at jeffhecht.com.