OPTICAL MATERIALS: Semiconductor-core fiber promises novel nonlinear fiber-based devices
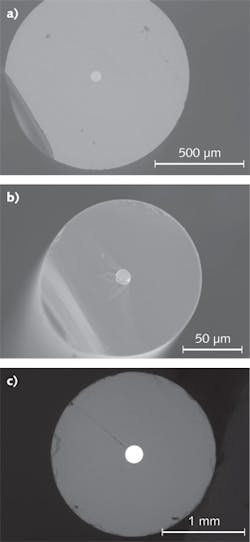
JOHN BALLATO, THOMAS HAWKINS, PAUL FOY, COLIN McMILLEN, ROGER STOLEN, and ROBERT RICE
Crystalline semiconductor core optical fibers are still in their infancy but are generating growing interest globally. Such fibers—which are currently focused on Group IV and III-V semiconductors—have the potential to marry the benefits of long, flexible, and robust fiber-based waveguides with the nonlinear, Raman, and infrared (IR) transparency of semiconductor planar waveguides.
Our initial successes in glass-clad semiconductor optical fibers fabricated using a molten core method include cores of crystalline silicon, germanium, and indium antimonide (see Fig. 1).1,2,3 In the molten core method, the semiconductor is placed inside a cladding glass tube. The cladding glass is chosen such that the semiconductor core is molten at the temperature where the glass cladding is drawn into fiber. In essence the cladding glass serves as a crucible to confine the semiconductor melt as the glass crucible draws directly into optical fiber.
Results
Though more a convenience for proof-of-concept studies, we used silica glass as the cladding for the silicon (Si) core fiber and DURAN, a commercial alkali borosilicate glass, for the germanium (Ge) core fiber. The silica draws at a temperature about 500K above the melting point of the Si. While a closer match between core melting point and cladding draw temperature would be preferable, silica is very strong, commercially available, and proves the concept. The DURAN draws at only about 60K above the Ge melting point, providing a better match. In all cases, we found oxygen and other cladding components in the core, likely resulting from dissolution of the cladding glass by the core melt. This is currently a limitation but several efforts are underway to mitigate the interaction.
Crystallinity and crystallography: In all cases and under all draw conditions, we found the semiconductor cores to be highly crystalline (see Fig. 2). The lack of other phases in the x-ray diffraction patterns suggests that the aforementioned oxides likely reside as amorphous precipitates.We have examined the nature of the semiconductor crystallography. An especially intriguing fundamental question is whether these crystalline core optical fibers contain significantly long regions of single crystallinity given the rapid quench rate associated with fiber draw. Further, what are their crystallographic orientations with respect to the fiber axis?
While each of the semiconductor optical fibers studied to date has been polycrystalline over its entire length (ranging from several to more than 200 continuous meters), the fibers do exhibit localized regions of single crystallinity. About 85% of the Ge fiber exhibited localized single crystallinity with a given crystallographic orientation persisting for lengths up to 15 mm before a new crystallographic orientation was encountered; quite remarkable for draw rates on the order of a meter per second. Crystallographic orientation of the semiconductor core, with respect to the fiber axis, always favored low index planes though a small angular offset was always present.4
Optical properties: The transmission through silica-clad Si core optical fibers at both 1.3 and 1.5 μm wavelengths was approximately 2.7 dB/cm, whereas at about 3 μm an attenuation of 4.3 dB/m was measured. The dominant extrinsic loss of the current optical fibers appears to be scattering from amorphous oxide particulates formed by dissolution of the cladding glass by the molten semiconductor. While there is a reasonable λ-4 trend in the measured attenuation, implying that Rayleigh scattering dominates, the near equivalency of losses at 1.3 and 1.5 μm suggests that other factors may influence the near-IR properties.
What might be a reasonable benchmark for practical attenuation given continued progress? At a wavelength of 3 μm, multiphonon and free carrier absorption are negligible in intrinsic Si, as is the loss of the silica cladding. As a result, extrinsic scattering and absorption are expected to dominate the attenuation of a large-core fiber. A reduction of the oxide content by a factor of 100 should lower the loss to 0.04 dB/m corresponding to an absorption coefficient of 10-4 cm-1, which is close to the lowest reported loss in Si. We have no current estimates of scattering from grain boundaries or absorption from other impurities, but it appears that 50 dB/km might be possible based on the intrinsic properties of Si and silica.
Future prospects
Crystalline core fibers—especially those fabricated by a molten core approach—raise several intriguing fundamental scientific questions. Conventional methods grow crystals at rates ranging from millimeters per day (such as hydrothermal) to millimeters per minutes (like laser heated pedestal growth, or LHPG). The molten core-derived crystalline semiconductor core optical fibers are fabricated at rates of meters per second. Such rates are about 103 times faster than LHPG and more than 106 times faster than hydrothermal, which begs the question: Where does thermodynamics end and kinetics take over?
The high degree of crystallinity and reasonably long-range crystallography implies that thermodynamics still dominates at these high quench rates. Based on the crystallization rates of amorphous Si, draw speeds on the order of 18 m/s might be possible for silicon optical fiber. This suggests that long continuous lengths can be made at industry-relevant manufacturing speeds.5
Applications
The ability to draw long lengths of crystalline semiconductor core optical fibers using commercially accepted manufacturing methods opens the door to unify long propagation distances with the optical confinement necessary to maintain high pump intensity, all in a fiber structure that provides better heat dissipation than do traditional bulk crystals.
The unary semiconductors are characterized by high linear refractive indices and large third-order χ3 nonlinearity. The Raman gain coefficient for silicon is approximately 104 times greater than that of silica glass and suggests that silicon core fibers might make excellent Raman amplifiers, particularly in the midwave IR (MWIR). This spectral band includes vibrational absorptions associated with the chemical precursors of weapons of mass destruction, and has very low solar and thermal background radiation. Further, the impact of atmospheric turbulence on the propagation of free-space laser beams is much less in this band than in the visible and near-IR. As a result, crystalline semiconductor core optical fibers can be quite useful as MWIR oscillators and amplifiers.
A Si or Ge fiber Raman amplifier could offer a dramatic reduction in fiber length and large increase in average output power. In Ge, the transmission window extends well past 10 μm, enabling beam-combining with carbon monoxide (CO), carbon dioxide (CO2), and longwave IR (LWIR) quantum cascade lasers. Germanium fibers might also be feasible as terahertz waveguides given the low loss of Ge in the terahertz spectral region.
The recent realization of a crystalline binary semiconductor (InSb) core optical fiber opens the door to new possibilities for nonlinear fiber-based devices. Compound semiconductors such as InSb, InP (indium phosphide), or GaAs (gallium arsenide) lack a center of crystallographic symmetry such that second-order χ2 optical nonlinearities could exist.
The general benefits of optical fibers based on crystalline semiconductor cores with glass cladding will be high gain, narrow gain spectra, and excellent thermal conductivity. This will enable short fibers for reduced nonlinear effects when desired (stimulated Brillouin scattering, stimulated Raman scattering, four-wave mixing, and so on), and could potentially reduce the cost of diode-pumped crystal host lasers drastically.
Conclusions
Through still young, the field of glass-clad semiconductor core optical fiber offers opportunities for both fundamental scientific insights and practical commercial impact. The molten-core approach shows great potential for realizing long continuous lengths of glass-clad crystalline semiconductor core optical fibers using practical and scalable production methods. The best fibers to date exhibit losses on the order of 2 dB/cm in the near-IR and about 4 dB/m in the mid-IR. Clearly not optimized, it seems possible—based on the intrinsic properties of semiconductors and the thermodynamic and kinetic influences of the fabrication process-that attenuations on the order of 50 dB/km could be achieved with continued effort. A shorter-term goal would be an attenuation of 1 dB/m as this would enable considerable opportunities for a wide range of IR and nonlinear optical devices.
ACKNOWLEDGMENTS
The authors wish to acknowledge financial support from Clemson University, the Northrop Grumman Corporation, the Raytheon Company, and the Defense Advanced Research Projects Agency (DARPA) under contract HR0011-08-C-0137.
REFERENCES
1. J. Ballato et al., "Silicon optical fiber," Opt. Exp., 16, 18675-18683 (2008).
2. J. Ballato et al., "Glass-clad single-crystal germanium optical fiber," Opt. Exp., 17, 8029-8035 (2009).
3. J. Ballato et al., "Binary III-V core semiconductor optical fiber," Opt. Exp., 18, 4972-4979 (2009).
4. C. McMillen et al., "On crystallographic orientation in crystal core optical fibers," Opt. Mat., 32, 862-867 (2010).
5. J. Ballato, T. Hawkins, P. Foy, R. Stolen, and R. Rice, "Advancements in Semiconductor Core Optical Fiber," Optical Fiber Technology, 16, 399-408 (2010).
John Ballato is professor of materials science and director of the Center for Optical Materials Science and Engineering Technologies (COMSET), Thomas Hawkins is a research associate, Paul Foy is director of the Optical Fiber Laboratories, Roger Stolen is distinguished visiting professor at the School of Materials Science and Engineering, and Colin McMillen is research associate in the department of chemistry at Clemson University, Clemson, SC 29634, USA; e-mail [email protected].Robert Rice is senior scientist (retired) at Northrop Grumman Space Technology, Redondo Beach, CA 90278, USA.