Fluorescence-microscopy technique has high photon-count rate per molecule
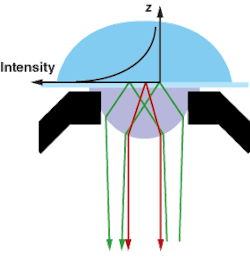
Spotting individual or small groups of molecules, and identifying them through spectroscopy, is becoming increasingly important in biological and medical research. Fluorescence-correlation spectroscopy (FCS), in which fluctuations in fluorescence are tracked by sensitive light detectors and statistically analyzed to understand molecular goings-on such as diffusion, can be combined with confocal techniques to sense molecules; however, a relatively large volume of analyte must be excited with this technique, which loses precision. Total-internal-reflection FCS (TIR-FCS) relies on evanescent light waves at an interface and is more precise, but suffers from low light-collection efficiency.
Researchers at The Ecole Polytechnique Fédérale de Lausanne (Lausanne, Switzerland) and the Universität Basel (Basel, Switzerland) have combined aspects of both these techniques into a version of TIR-FCS that has high light-collection efficiency and a small observation volume.1 A 100× objective lens with a numerical aperture (NA) of 1.45 is combined with a prismless approach to evanescent illumination; the returning fluorescence is collected by the same objective and coupled into a fiber, at the other end of which is a single-photon avalanche photodiode (SPAD). The signal from the SPAD is sent to a single-photon-counting module, which produces a temporally precise rendering of intensity versus time.
A glass cover slide carrying the sample is placed directly against the objective’s last element. Argon-ion-laser excitation light at 488 nm is sent through the objective outside its normal NA; the result is that, at the glass-sample interface, the excitation light undergoes TIR-creating an evanescent wave in the sample-and reenters the objective (see figure). A pivoting glass plate in the optical train allows precise lateral control of the excitation beam, and thus proper positioning just outside the objective’s normal NA range. A pinhole in the microscope’s image plane defines the observation volume.
The researchers detected and monitored individual dye (rhodamine 6G) molecules adsorbing and desorbing on a glass cover slide after diffusing through a sample. First, they used a conventional confocal FCS system to ensure that the molecules were not clumping together. In their new setup, they then monitored intensity bursts, setting the threshold for a burst at 10 counts per 100 µs, against a background of 2 counts per 100 μs. They detected an average of 7 bursts/s, with an overlap of two or more bursts occurring an average of once per 10 s. The highest single bursts had counts of at least 70, resulting in a signal-to-noise (S/N) ratio of 35 for those counts.
Next, freely diffusing dye molecules were observed; they were prevented from adhering to the cover slide by treating the slides with oxygen plasma before use, rendering them hydrophilic. In this case, no intensity bursts were observed, but autocorrelation curves from the time trace were determined, which allowed diffusion coefficients to be determined and compared with a model. In this case, S/N was about 39.
The experimental TIR-FCS data for freely diffusing fluorophores agreed well with numerical calculations. Although a single-point detector was used in the setup, a camera could be connected to the microscope to allow TIR-fluorescence imaging as well.
REFERENCE
1. Kai Hassler et al., Optics Express13(19) (Sep. 19, 2005).
About the Author
John Wallace
Senior Technical Editor (1998-2022)
John Wallace was with Laser Focus World for nearly 25 years, retiring in late June 2022. He obtained a bachelor's degree in mechanical engineering and physics at Rutgers University and a master's in optical engineering at the University of Rochester. Before becoming an editor, John worked as an engineer at RCA, Exxon, Eastman Kodak, and GCA Corporation.