Advanced Optics: Advanced fiber optics further biomedicine
The application of optical fiber usage in biomedicine has gained momentum in recent years. Historically, optical fiber has been used primarily for endoscopy and laser power delivery, but now a wide base of academic and corporate-funded research is exploring both in vivo and in vitro areas, with a number of new applications entering full-scale manufacturing.
This growth has been fueled by a list of capabilities unmatched by any other single material, including electromagnetic interference (EMI)-free transmission of energy and signals; small diameter; biocompatible coatings; high mechanical flexibility; image transmission; and the ability to sense pressure, temperature, chemicals, and strain. Consequently, optical fibers have found their way into a wide range of applications, including:
- Chemical sensing
- Fluorescence microscopy
- Haptic feedback for laparoscopy
- Laser ablation
- Endoscopy
- Hair removal
- Spectroscopy
- Single-fiber endoscopy
- Laser eye surgery
- Optical coherence tomography (OCT)
- DNA/RNA sequencing
- In vivo pressure sensing
- 3D catheter shape sensing for angioplasty
Such a broad range of applications requires a wide selection of optical fibers with designs ranging from large-core, large-diameter multimode (MM) fibers for high-power laser light delivery to small-core, reduced-diameter single-mode (SM) fiber for in vivo sensing. Additional requirements demand advanced coatings, as standard optical fibers have an acrylate coating that adds significant diameter to the overall fiber size and is suitable up to 85°C. Specialty coatings, on the other hand, enable reduced thickness and greater tolerances. Polyimide, for instance, can withstand temperatures up to 300°C as well as autoclave sterilization.
Mechanical properties
Each fiber design offers not only different optical properties, based on core size and composition, but also different mechanical properties, based on the combined composite structure of the core, cladding, and coating. And for in vivo applications, device miniaturization is required: The optical fiber typically needs to fit within a catheter tube where typical sizes for surgical procedures, such as coronary angioplasty, range from 5F to 8F (1473–2286 µm diameter).
Although even a standard (non-specialized) optical fiber with a 245 µm coating diameter can easily fit within this space, a catheter will typically need to accommodate other devices as well, including guide wires and manipulation tools—so reduction of cladding and coating diameters are typically required. Specialty optical fiber suppliers commonly manufacture fibers with reduced coating diameters, even down to 70 µm or below, on scale with the diameter of a human hair (see Fig. 1).
Beyond dimensions, an optical fiber’s stiffness must be considered as it can impact the flexibility of the overall catheter assembly, affecting the ability to guide the catheter correctly. The stiffness of an optical fiber is determined mainly by the diameter of the cladding and the bulk material, typically silica, which approximates the rigidity of soft metals such as aluminum.
Signal considerations and sensing
Optical fibers do not contain any electrically conductive or magnetic materials and can be considered free from electromagnetic interference (EMI). Thus, optical fibers can be used in magnetic resonance imaging (MRI) devices and in close proximity to radio frequency (RF) electrosurgery tools used for cutting, coagulating, desiccating, and fulgurating tissue.
Optical fibers are inherently sensitive to pressure, temperature, strain, and chemicals, and thus offer an outstanding solution for applications involving these measurands. Alternative technologies typically require an electrical sensor element, making them not only unsuitable for MRI and RF use, but also requiring a separate signal line for each sensor element, which adds to the cross-sectional area.
By contrast, an optical fiber can have thousands of different, uniquely identifiable sensor elements and still require only the single line of fiber, without additional sacrifice to the diameter. This characteristic enables simultaneous transmission of multiple messages along one channel of communication—an arrangement known as multiplexing—which often uses specially modified regions of the core called fiber Bragg gratings (FBGs). Multiplexing is achieved either by allowing each sensor to have a different sub-section of the light source’s spectral range, known as wavelength division multiplexing (WDM), or else by having each sensor as an identical element but spatially separated such that the time for the light to reach each sensor is different, known as time division multiplexing (TDM).
Next-gen applications
One of the most impressive up-and-coming biomedical applications is that of 3D shape sensing to facilitate procedures such as coronary angioplasty with minimal use of x-ray-assisted guidance. In such procedures, an initial x-ray image is required but the live updates of the position of the guide wire are provided by the optical fiber. This is analogous to GPS guidance for vehicles, with the pre-loaded map being provided by the x-ray device and the GPS signal corollary coming from the optical fiber’s feedback.
The technical premise is based on the concept that because an optical fiber exhibits strain when bent into different shapes, it is possible to design the optical fiber such that it can measure and differentiate these strains to allow accurate reconstruction of the shape of the optical fiber. This application is enabled by a highly complex optical fiber (see Fig. 1).
In vivo pressure sensing has seen a flurry of activity in recent years using optical fibers either point-sensing elements or as distributed “maps” of pressure variation along the length of the optical fiber. This latter capability is achieved through use of a twin-hole optical fiber, which responds to external pressure asymmetrically and creates an optical effect called birefringence, which is sensed in the fiber’s core (see Fig. 2).Historically, 3D shape sensing and distributed in vivo pressure sensing were not feasible, but these new optical fibers have made them possible—and dramatically reduced the barrier to entry for new designers.
Fiber optic probes
Fiber optic probes have traditionally been developed and sold primarily for high-power laser applications. But a new generation of probes allows both output of light in a particular format and collection of reflected light back into the optical fiber. This capability allows fiber to be used as a send-and-receive type element for applications such as in vivo optical coherence tomography (OCT) and fluorescence microscopy.
Normally, light exits a fiber longitudinally, along the axial length. However, it is possible to create a probe such that the light is turned 90° at the tip of the fiber, which enables illumination and analysis of the side walls of any cavity, for example the bronchus (see Fig. 3). This enables imaging of surface and sub-surface structures as well as simultaneous surface chemical detection—for applications such as early cancer diagnosis.A probe consisting of a single optical fiber enables a millimeter-scale endoscope product, which translates to a procedure that is far less challenging to the patient, far less risky, and far less costly than one done with a large-diameter white-light endoscope. This, in principle, increases the potential uptake to allow the procedure to be used more readily as a simple screening process.
Fiber specialists hold key knowledge
Recent advances in optical fiber manufacturing has enabled production of a wide variety of optical fibers capable of addressing a new range of biomedical applications (see Fig. 4). Historically, optical fiber developments have originated through physics-oriented organizations. But now, biomedical applications development is driven by organizations focused on specific areas in chemistry and biology, often without academic and engineering background with optical technologies.For this reason, technical support provided by the specialty fiber manufacturer is critical for ensuring successful and timely integration of optical technologies into biomedical devices. Leveraging this know-how, gained through decades of practice, can substantially reduce development time of new biomedical devices: Optical fiber specialists can help steer clear of known pitfalls, and enable initial design-in of suitable options rather than progressing through iterative improvements as the best fiber is identified. With such cooperation and the growing optics knowledge base within biomedicine, biomedical applications are expected to keep growing with new, diverse products increasingly emerging.
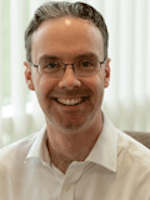
Andy Gillooly | Business Development Manager, Fibercore
Andy Gillooly is the business development manager at Fibercore (Southampton, U.K.).
In 2001, Andy graduated from the University of Birmingham, with a master’s degree in physics and received a PhD in fiber Bragg gratings (FBGs) from Aston University, England.
He spent over four years as a senior development engineer at SPI Lasers, developing FBGs and pulsed fiber lasers. After SPI, Andy joined Sharp Laboratories of Europe, responsible for the optical design and technical customer interactions for Sharp’s wafer-level camera project. Later, Andy joined Fibercore, where he is currently responsible for driving the company forward into new markets with new products; he has currently been with Fibercore for 14 years.