IMAGING: Lensless ghost imaging uses true thermal light
Ghost imaging is as spooky as it sounds, and is indeed related to “spooky action at a distance” and quantum-entangled photons. Research initiated in 1995 by University of Maryland (College Park, MD) professor Yanhua Shih showed that if one of a pair of entangled photons passed through a pattern in a mask to trigger a detector, and another photon was captured by a second detector, the coincidence counts between the two detectors could reveal an image of the pattern—a process called correlated two-photon imaging. Essentially, the image is called a “ghost image” because it is formed by light that never interacts with the object. The technology has numerous potential applications in imaging objects through clouds, smoke, or other obscuring items.
Ghost imaging can also be achieved using a thermal light source. In this case, reflected and scattered light from an object is detected by second-order correlation techniques. But in all lensless demonstrations using this method so far, a pseudo-thermal light source was used, consisting of a laser passing through a rotating ground-glass plate to increase its coherence time.
Now, researchers at the Chinese Academy of Sciences (Beijing, China) have achieved what they believe to be the first demonstration of lensless ghost imaging using a commercially available true thermal light source—a hollow-cathode lamp operating at the neon wavelength of 692.9 nm with a measured coherence time of 0.1 ns (much shorter than pseudo-thermal sources).1 Here, “lensless” means that no lens is used to image the object.
The demonstration is significant because there are many kinds of true thermal light sources at wavelengths where lenses are impractical or where no laser radiation (used to generate a narrow-linewidth pseudo-thermal light) is available, such as x-rays, gamma rays, and terahertz sources.
Seeing the ghost
The experimental setup used to obtain the ghost image of a mask consisting of two holes poked in a copper foil is quite simple. The hollow-cathode lamp illumination passes through a monochromator to extract the approximately 693 nm neon spectral line. This light is then passed through a beamsplitter and half of it passes through the copper foil and then through a series of collimators to a single-photon detector called a “bucket” detector because it collects all the transmitted light without providing any spatial information. The other half of the light from the source is sent directly to a second single-photon detector that is scanned and only receives the light arriving at a given spot.
The output signals from the two detectors are then sent to a time-to-amplitude converter (TAC); the first and second detectors, respectively, provide the “start” and “stop” signals for the TAC. A multichannel analyzer can then use the TAC information to build a histogram of the coincidence counts (both photons received simultaneously) as a function of the difference in the times of arrival of the two photons at the different detectors.
From this coincidence histogram and distance parameters of the object and image planes within the experimental setup, the transverse normalized second-order correlation function, Δg(2), can be computed (see figure). This function is then mathematically translated into a spatial representation or ghost image of the illuminated object.
Research professor Ling-An Wu at the Institute of Physics, Chinese Academy of Sciences, says, “In the not-too-distant future, we may expect to see high-resolution ghost imaging of microscopic biological samples with x-rays or even atoms and fermions, as well as of macroscopic objects under surveillance with free radiation from the Sun.”
REFERENCE
- Xi-Hao Chen et al., Optics Letters 34(5) p. 695 (March 1, 2009).
About the Author
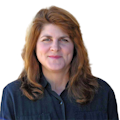
Gail Overton
Senior Editor (2004-2020)
Gail has more than 30 years of engineering, marketing, product management, and editorial experience in the photonics and optical communications industry. Before joining the staff at Laser Focus World in 2004, she held many product management and product marketing roles in the fiber-optics industry, most notably at Hughes (El Segundo, CA), GTE Labs (Waltham, MA), Corning (Corning, NY), Photon Kinetics (Beaverton, OR), and Newport Corporation (Irvine, CA). During her marketing career, Gail published articles in WDM Solutions and Sensors magazine and traveled internationally to conduct product and sales training. Gail received her BS degree in physics, with an emphasis in optics, from San Diego State University in San Diego, CA in May 1986.