Photonics Products: Fiber Lasers: Visible fiber lasers do red, green, and now bluish
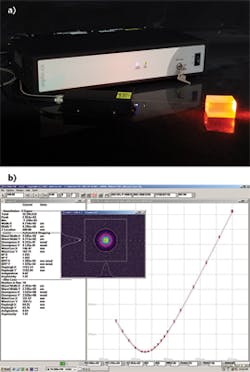
Visible-wavelength fiber lasers (often called visible fiber lasers) have, over the past decade, established their presence in the commercial laser arena. But what is a visible fiber laser? The question is an interesting one, because there is actually no fiber laser on the market that produces visible laser light from within the lasing fiber itself.
Visible light can, however, be obtained from a near-infrared (IR)-emitting fiber laser by external frequency conversion—for example, Raman-shifting, frequency-doubling, frequency sum-mixing, or combinations of these approaches. For the purposes of this article, this will be the definition of visible fiber laser. Note that direct-diode lasers, where light from laser diodes is coupled into a non-gain fiber, do not fall under this definition.
Visible fiber lasers come in continuous-wave (CW) and pulsed form, range from low-power to a hundred or more watts in output, and have uses ranging from science to industry (especially materials processing) to general laser use.
Lasers for science and medicine
The origins of visible-fiber-laser technology are as varied as the companies that produce these devices. For example, MPB Communications (MPBC; Montreal, Quebec) supplies Raman amplification subsystems to the fiber-optic communications industry. In 2001, when the telecom bubble burst, MPBC sought to diversify its markets. With the company’s store of Raman technology, the field of fiber lasers was a natural path, according to Claudette Linton, business development, visible fiber lasers.
“By reproducing MPBC’s telecom Raman fiber lasers, but now using polarization-maintaining fibers and components, MPBC was able to frequency-double the fundamental fiber-laser wavelengths into the visible with a single-pass periodically poled lithium niobate (PPLN) second-harmonic generator,” Linton says. “By utilizing this straightforward frequency-doubling method, the excellent beam characteristics of fiber lasers is not compromised.”
MPBC launched the first visible fiber laser at a 560 nm wavelength at SPIE Photonics West in 2006, Linton says. The laser was soon adopted for flow-cytometry applications by William Telford at NIH National Cancer Institute (Bethesda, MD). With MPBC’s internal fiber-Bragg-grating facility, the company is able to quickly adapt recipes to generate new wavelengths in response to specific requirements, Linton adds. MPBC currently produces visible fiber lasers with 26 commercial wavelengths from 488 to 775 nm and with powers ranging from 0.2 to 5 W, depending on the application needs.
The MPBC lasers have now been integrated into commercial superresolution microscopy systems, including stimulated emission depletion (STED), gated STED, stochastic optical reconstruction microscopy (STORM), lightsheet, and so on; flow cytometry; and DNA-sequencing platforms that to date have accumulated more than 50,000 hours run time, showing the reliability of fiber laser technology, Linton says.
The company has collaborated with researchers including Vladislav Verkhusha (Department of Anatomy and Structural Biology at the Albert Einstein College of Medicine), Nobel Laureate Stefan Hell (Max Plank Institute), and Nobel Laureate Eric Betzig (Howard Hughes Medical Institute; HHMI). The wavelengths sought by these researchers were often difficult to achieve and/or to power-scale with diode technology. For example, in 2017, Betzig requested a 607 nm wavelength and 1 W of optical power for a new lightsheet microscopy platform. Diode-pumped solid-state (DPSS) lasers were unable to achieve the power levels required for the application, and high-power optically pumped semiconductor lasers (OPSLs) were unable to provide the beam quality necessary for the application. Within a short time frame, Linton says, MPBC designed, tested, and began shipment of a 607 nm fiber laser that has >1 W of output power and a beam quality M2 <1.1 (see Fig. 1).
Betzig wanted the 607 nm wavelength to optimally excite the bright and photostable fluorescent protein mCardinal, which is sufficiently separated spectrally from orange-red fluorescent proteins such as mApple or tdTomato that two-color imaging (orange-red and deep red) at less phototoxic red wavelengths becomes an option, or three-color imaging with the inclusion of a green fluorescent protein presents an option as well. The laser is now under test at HHMI and has been provided to collaborators who are working in partnership with HHMI.
MPBC is expanding its visible-fiber-laser line to include high-power single-frequency fiber lasers and single-frequency Raman fiber amplifiers used for atom cooling, holography, and guide-star lasers, and high power subnanosecond pulsed visible fiber lasers used for STED. “Superresolution microscopy was a novel technique when we first started,” says Jane Bachynski, MPBC’s president. “Now every major microscopy company has their own superresolution platform, enabled by the visible fiber laser due to its performance.”
Specialty fibers extend visible emission to the blue
Azurlight Systems (Pessac, France) designs and manufactures visible-wavelength fiber lasers by frequency-doubling fundamental IR fiber lasers, as described by Pierre Laygue, the company’s director of sales and marketing. Specific specialty fibers have been developed that have extended ytterbium (Yb) gain bandwidth down to 976 nm (1030 nm being the traditional lower limit). This has enabled the company to open up visible-wavelength coverage into the blue region of the optical spectrum (see Fig. 2).“The conversion takes place in a specific crystal and with a single-pass architecture, with optimized crystal length and technology to match the IR wavelength and power so that its conversion efficiency is maximum,” Laygue says. “The single-pass architecture allows conservation of all main properties of the IR light (robust single-frequency operation, intensity noise close to shot noise, perfect mode quality, and extremely good pointing stability).”
The main applications of Azurlight’s high-power (2 W at 488 nm and 10 W at either 515 or 532 nm) visible-wavelength fiber lasers are argon-ion (Ar+) laser replacement, laser Doppler velocimetry (LDV), holography, interferometry, superresolution microscopy, and pumping of optical parametric oscillators (OPOs) and solid-state lasers.
“Solid-state replacement technology for the 488 nm Ar+ line [which has a cyan-blue color] has been notoriously difficult to develop, mainly because of the difficulty of exploiting the three-level transition of the Yb3+ ion at 976 nm,” Laygue says. A 976 nm 10 W fiber laser using the company’s specialty fiber is the basis for the 488 nm fiber laser, he notes. “The result is a product with the highest available power at 488 nm, with mode quality and pointing stability greatly superior to Ar+ lasers,” he says. “The target market for this source is replacement of Ar+ lasers by eliminating the recurrent and expensive refurbishment, eliminating water cooling from the lab, and improving optical beam characteristics such as pointing stability and output power stability.”
In one example, companies designing LDV systems recouple the laser light into fibers that deliver the beam close to the experimental volume. Argon-ion laser beam pointing being inherently unstable, these companies have to readjust the positioning sometimes several times a day. “With our 488 nm fiber laser, the pointing stability is so good that the coupling system can remain untouched for months,” Laygue says.
Properties of the EVERESTnano AP-515 green pulsed laser | |
Parameter | Specification |
Operation mode | Pulsed |
Operating wavelength | 515 nm (green) |
Average power | 10 W, 20 W, 30 W, 50 W |
Pulse width | 5 ns |
Pulse energy | 100 µJ |
Beam quality, M2 | <1.2 |
Output power stability | Within ±5% |
Output delivery | Collimated output beam |
Pulsed green for materials processing
Fiber lasers in general have deep connections to materials processing, and this goes for visible fiber lasers, too. In addition to IR fiber lasers, AdValue Photonics (Tucson, AZ) produces a line of green-emitting pulsed fiber lasers for materials processing that emit either nanosecond (EVERESTnano Green) or picosecond (EVERESTpico Green) pulses at high average powers. Both lasers emit at 515 nm and are based on a 1030 nm master-oscillator power-amplifier (MOPA) pulsed fiber laser with frequency doubling in free-space geometry for green wavelength generation.
For example, the nanosecond-pulse version produces pulses with 5 ns duration and 100 µJ energy—pulse-repetition rates of 100, 200, 300, or 500 kHz result in average powers of 10, 20, 30, or 50 W (see table). The picosecond laser produces pulses with 50 ps duration at average powers of 10, 15, 20, or 30 W. Both lasers are water-cooled, with a chiller as an option.
According to Katherine Liu, director of marketing and sales at AdValue Photonics, target applications for these green lasers include drilling, cutting, ablating, and scribing, with target materials including (but not limited to) glass, sapphire, ceramics, silicon, printed circuit boards (PCBs), metals, composites, and polymers.
A visible fiber laser is an advantage for processing of materials that have a higher absorptance in the visible than in the IR. Some metals, such as copper and gold, have an absorptance several times higher in the green than at the fundamental IR wavelength. Copper in particular is widely used in electronics.
“The AdValue Photonics’ laser technology allows high pulse energy and short pulse width from a fiber-based pulsed green laser, as well as constant pulse energy and pulse width at different pulse repetition rate,” Liu says. “In addition, the technology allows very high pulse repetition rates into the megahertz regime.”
One application example noted by Liu is glass drilling and cutting. “The high-repetition-rate nanosecond green fiber laser meets the increased demands on high-quality and high-efficiency transparent material processing,” she explains. “Multiple laser-processing techniques such as laser drilling, cutting, scribing, and marking are widely applied to versatile application areas. For example, by using the laser-trepanning drilling process, zero-tapered holes with chipping size down to 50 µm and an equivalent cutting speed up to 22 mm/s for a 0.5-mm-thickness wafer are achieved. From soda lime to quartz glass, various transparent materials can be machined selectively and digitally via noncontact processing.”
A sampling of visible fiber lasers
Numerous fiber-laser makers produce visible fiber lasers as part of their lineup. A complete list of companies and products is beyond this article, but a few more examples follow.
As part of its PyroFlex line, Eolite Systems (Pessac, France) makes a green-emitting pulsed fiber laser for materials processing that has the unusual ability to allow arbitrary pulse shaping capability, detailed control over precise pulse shape and complex pulse trains, with 1 ns resolution. The laser has a maximum average power of 10 W, maximum pulse energy of 100 µJ, and a beam quality M2 <1.3.
IPG Photonics (Oxford, MA) makes a wide variety of visible fiber lasers, including green-emitting CW fiber lasers with output powers of 0.5 to 100 W and visible CW Raman fiber lasers with wavelengths ranging from 515 to 603 nm, and output powers from 1.5 to 15 W. The company also produces a luminaire laser system (RGB-6P/3P) for laser cinema that combines red- and green-emitting fiber lasers with direct-diode blue emitters (the latter of which is not a true visible fiber laser, but is instead another very useful path towards visible fiber-based light sources).
Optromix (Cambridge, MA) has a line of tunable fiber lasers that includes a single-mode CW visible fiber laser tunable over a wide range in the green (515–562 nm). The linewidth of the laser can be tailored by the company for the user from options of 0.01, 0.05, 0.1, and 0.3 nm. Outputs of the various models in the lineup range from 100 mW to 6 W, all with beam quality M2 of better than 1.1. Applications include biotechnology, spectroscopy, and holography.
DISCLAIMER: While we try to include information from the broadest possible number of companies that manufacture the products featured in our Photonics Products series, because of limited word count as well as deadlines that cannot always be met by requested contributors, we cannot possibly include all companies and regret if your company is not included in our series.
For More Information
Companies mentioned in this article include:
AdValue Photonics
Tucson, AZ
Azurlight Systems
Pessac, France
Eolite Systems
Pessac, France
IPG Photonics
Oxford, MA
MPB Communications
Montreal, QC, Canada
Optromix
Cambridge, MA
About the Author
John Wallace
Senior Technical Editor (1998-2022)
John Wallace was with Laser Focus World for nearly 25 years, retiring in late June 2022. He obtained a bachelor's degree in mechanical engineering and physics at Rutgers University and a master's in optical engineering at the University of Rochester. Before becoming an editor, John worked as an engineer at RCA, Exxon, Eastman Kodak, and GCA Corporation.