Optics and photonics enable the fight against COVID-19
COVID-19 had a more considerable impact on day-to-day life this past year than any other event in recent history. As the pandemic continues to grip the world, the general public has been following the latest developments in testing, treatment, disinfection, and vaccine development closer than ever before. Surprisingly, with all the media attention, little if any attention was on the fact that many of these advancements would not be possible without photonics.
The phrase “photonics is an enabling technology,” so often stated within the community, could not be more apropos when it comes to COVID-19 since biomedicine is undoubtedly one of the sectors most heavily affected by photonics. In fact, one could argue that no single industry has been influenced more heavily by photonics than healthcare, with the possible exception of telecommunications. As a result, we have decided to take a deep dive into how photonics technologies are applied to help combat the COVID-19 pandemic. This article explores how photonics is currently applied in COVID-19 testing while simultaneously looking at how photonics is fueling the next generation of technologies on the horizon for 2021.
The role of fluorescence in COVID-19 testing
For diagnosing active infections of COVID-19, the primary testing protocols involve using a process known as reverse-transcription polymerase chain reaction (rt-PCR). Since PCR is a method of exponentially amplifying DNA, it’s valuable first to review a DNA molecule’s basic structure to better understand how the process works. DNA is a double-stranded molecule consisting of four nucleic acids: adenine (A), cytosine (C), guanine (G), and thymine (T). Adenine can only bind to thymine, and guanine can only bind to cytosine to form base pairs, producing the 4-bit genomic code that facilitates DNA self-replication.
In traditional PCR, a strand of DNA is denatured, causing it to “unzip” and split into two halves. Next, a polymerase is added along with a DNA-coded primer designed for the genome of interest. The polymerase initializes a chemical process by which the two halves of the DNA strain are each “filled in” with the appropriate nucleic acid, creating two complete DNA molecules. This process is repeated, creating four molecules, then eight, then 16, and so on, exponentially increasing the total amount of DNA. This amplification only takes place if the base DNA sequence matches that of the primer, making it a highly selective measurement technique to confirm the presence of a particular antigen, like SARS-CoV-2, the virus that causes COVID-19. It should be noted that since coronavirus is a single-stranded RNA virus, additional steps must be taken first to convert the RNA to DNA hence the need for reverse transcription, but the result is still the same.
A small RNA probe with a fluorophore linked to one end and a quenching molecule attached to the other end is used to tag the target DNA. The presence of the quenching molecule deactivates the fluorophore when it is nearby, rendering it inert in solution, but after bonding with the target RNA, the polymerase will cause the fluorophore to be released and activated (see Fig. 1). As the PCR process repeats, the fluorescence signal will eventually become strong enough to be detected.1 Therefore, every PCR testing system requires an excitation source (typically an LED), a photodetector, and multiple optical filters.
Antibody detection is also a powerful tool for determining if someone was previously infected and tracking vaccination efficacy. Currently, enzyme-linked immunosorbent assay (ELISA) is the gold standard for antibody testing. An ELISA assay consists of an antigen immobilized via an antibody bound to a substrate, typically located in the well of a microplate array. When exposed to the sample, if antibodies are present in the serum, they will also bind to the antigen. A secondary antibody conjugated to a reporter molecule is then added to the well to allow for detection using either colorimetry or fluorescence. Therefore, ELISA testing systems require similar optical components as in rt-PCR testing systems.
As a result, every rt-PCR antigen test and ELISA antibody test for COVID-19 are not just tangentially dependent on photonics, but instead, at its core, fundamentally an optical analysis.
Developing point-of-care rapid screening
The value of ELISA and PCR testing cannot be understated, but unfortunately, neither method is well suited to rapid point-of-care (POC) testing. For example, even though ELISA is typically performed in a 96 well plate, allowing a large number of samples to be tested simultaneously, the total measurement time can still take several hours due to sample incubation times. Luckily, as was discussed in detail in the August 2020 issue of Laser Focus World,2 even before the current pandemic, modern spectroscopic techniques have been opening up pathways to POC medical diagnostics. Two of the most promising POC screening methods are surface plasmon resonance (SPR) sensors and surface-enhanced Raman spectroscopy (SERS) immunoassays.
POC antibody detection with SPR sensors
Surface plasmons are surface waves generated by coherent oscillation of conduction band electrons along the interface between a metal and a dielectric. As a result, surface plasmons are extremely sensitive to changes in a material’s dielectric constant, which is equal to the square root of the index of refraction. SPR sensors can, therefore, rapidly measure the changes in dielectric constant by refractometry, interferometry, or spectroscopy.
SPR sensors are constructed by depositing a thin noble metal film (such as gold, silver, or copper) on a glass substrate, and then an antigen can be bound to the metallic surface using a similar biochemical structure as described for ELISA. As antibodies attach to the antigens on the sensor’s surface, the dielectric constant slightly changes, resulting in a change to the resonant frequency of the surface plasmons. Therefore, SPR can provide rapid label-free detection since there is no need for secondary conjugation.
Figure 2 shows a schematic representation of an SPR sensor based on laser refraction. While this diagram is useful for developing a simplistic understanding of the process, broadband spectroscopy is a far more common methodology for measuring the refractive index change. Since spectra will have a dip in the spectrum corresponding to destructive interference when the incident light is in residence with the surface plasmons, the local minima of the transmission spectrum will shift as the sensor collects more and more analyte, allowing for extremely sensitive quantitation.POC antigen detection with SERS assays
Surface-enhanced Raman spectroscopy (SERS), which is an enhancement technique based on the coupling of local surface plasmon resonance (LSPR) and Raman scattering, is now one of the leading candidates being investigated as a possible solution for rapid COVID-19 screening. LSPR relies on nanostructured noble metals to locally increase the surface charge density to amplify the intensity of the surface plasmons, enhancing the intensity of the Raman scatter by up to a factor of 106. This extreme sensitivity, combined with Raman spectroscopy’s innate specificity, has made SERS a leading candidate for rapid POC COVID-19 screening.
While some teams are working on direct detection of the SARS-CoV-2 chemical fingerprint using Raman spectroscopy, challenges associated with ensuring the antigen is close enough to the nanoparticles or nanostructured substrate while simultaneously reducing the Raman scatter from smaller molecules in the sample make it nearly impossible to produce a repeatable test. As a result, a more typical approach is to use a SERS sandwich immunoassay, similar to that used in ELISA.
Traditional SERS sandwich assays are created by first binding antigen-specific antibodies to a solid gold substrate. Then, SERS nanoparticles are functionalized with the same antibodies and a highly Raman active reporter molecule, after which a four-step process occurs where first the substrate is exposed to a sample and then the nanoparticles are added, followed by a washing step, and then finally exposure to the laser. If the sample contains the viral antigen, it will serve as a linker binding the functionalized nanoparticles to the substrate, and the Raman spectrum of the reporter molecule is detected.4 While this process is extremely powerful, it limits field deployability because the washing step requires microfluidic capabilities. This process is even more challenging for noninvasive testing due to the high viscosity of saliva.
Bio-Stream Diagnostics (Edmonton, AB, Canada) has developed a SERS immunoassay where the solid substrate is replaced with a paramagnetic nanoparticle (PMP). This approach dramatically reduces the system’s complexity since the gold nanoparticles (AuNP) and the PMP can be stored in the same solution, eliminating the need for microfluidics and allowing a saliva sample to be in the vial, agitated, and then concentrated using a magnetic field. Figure 4 shows a schematic representation of the Bio-Stream sandwich assay, where the SARS-CoV-2 antigen is sandwiched between the antibody functionalized PMP and AuNP covalently bound to a reporter molecule.This assay was created using proprietary SARS-CoV-2 specific antibodies developed by Slavin’s supervisor Horacio Bach, a senior research scientist at Bio-Stream Diagnostics and Adjunct Professor/Manager of the IIRC Proteomic and Antibody Engineering Facility at the University of British Columbia. While unpublished, the results of their work have shown such promise that Bio-Stream Diagnostics has currently commissioned five prototype POC Raman systems to be developed for deployment testing PCR facilities across Canada to begin collecting comparative data.
For the sake of transparency, it should be noted that the author of this article is currently a consultant for Bio-Stream Diagnostics’ research and engineering teams, leading to the knowledge of the unpublished results discussed above.
Final thoughts
For brevity, we only focused on the application of photonics in COVID-19 testing. Still, it is essential to understand that photonics also plays a critical role in many other aspects of the fight against COVID-19, from UV-C disinfection systems5,6 to photodynamic therapy.7 The initial sequencing of the SARS-CoV-2 genome would not have been possible without fluorescence-based DNA sequencing. In correspondence with Jean-François Masson, he pointed out that “15 years ago, it would have taken weeks to decipher the sequence and 25 years ago, it would have taken years.” Masson went on to say, “Only because of next-generation sequencing were we able to react so quickly with vaccines, therapies, and other mitigation measures.” With all of this in mind, there is no doubt that photonics will continue to enable not only the next-generation technologies for this current pandemic, but will play a critical role in combating pathogens for the foreseeable future.
REFERENCES
1. L. J. Carter et al., ACS Cent. Sci., 6, 5, 591–605 (2020).
2. R. Chimenti, “Laser-based point-of-care testing and biomodulation therapy have a bright future,” Laser Focus World, 56, 8, 30–32 (Aug. 2020); https://bit.ly/LFW-Chimenti.
3. A. Djaileb et al., ChemRxiv (2020); https://doi.org/10.26434/chemrxiv.12118914.v1.
4. R. Thomas, K. A. Bakeev, M. Claybourn, and R. Chimenti, Spectroscopy, 28, 9, 2–8 (2013).
5. Y. Gerchman, H. Mamane, N. Friedman, and M. Mandelboim, J. Photochem. Photobiol. B: Biol., 212, 112044 (2020).
6. M. G. Strakhovskaya, G. A. Meerovich, A. N. Kuskov, S. A. Gonchukov, and V. B. Loschenov, Laser Phys. Lett., 17, 9, 093001 (2020).
7. N. Kipshidze, N. Yeo, and N. Kipshidze, Nat. Photonics, 14, 11, 651–652 (2020).
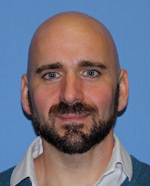
Robert V. Chimenti | Director, RVC Photonics LLC
Robert V. Chimenti is the Director of RVC Photonics LLC (Pitman, NJ), as well as a Visiting Assistant Professor in the Department of Physics and Astronomy at Rowan University (Glassboro, NJ). He has earned undergraduate degrees in physics, photonics, and business administration, as well as an M.S. in Electro-Optics from the University of Dayton. Over a nearly 20-year career in optics and photonics, he has primarily focused on the development of new laser and spectroscopy applications, with a heavy emphasis on vibrational spectroscopy. He is also very heavily involved in the Federation of Analytical Chemistry and Spectroscopy Societies (FACSS), where he has served for several years as the Workshops Chair for the annual SciX conference and will be taking over as General Chair for the 2021 SciX conference.