The origins of flow cytometry date back to the late-1940s with electrical engineer Wallace H. Coulter’s development of a technology that could count, classify, detect, and analyze the chemical and physical characteristics of cells and particles in fluid as they moved. It was a breakthrough that prompted Wolfgang Göhde, a researcher at the University of Münster (Germany), to establish flow cytometry by creating the first fluorescence-based flow cytometry device in the late-1960s. It was a game-changer.
Initially, flow cytometry—originally termed pulse cytophotometry—could count and analyze a small amount of cells with just a few parameters. Today, this technology can analyze thousands of cells (measured with more than 20 parameters) rapidly in real time, producing highly accurate data. It’s ideal for applications in biomedical research—specifically, where accuracy is crucial.
Moving past standard flow
Standard flow cytometry technology is a powerful cell analysis tool capable of detecting, imaging, and analyzing fluorescent probe-labeled cells as they flow past single or multiple lasers while suspended in a buffered salt-based solution. This process allows cell populations to be examined and/or purified based on their fluorescent or light-scattering characteristics. Flow cytometry is a swift process providing measurable, multiparametric analysis of cell populations at the single cell level (see Fig. 1).
Standard flow cytometry mainly provides information about surface and intracellular antigens—as well as about the nucleus and cell size and shape. It is used in various fields of medical and biological research as an effective means for studying the immune system and its response to infectious diseases.
The fluorescent markers are used to distinguish different cell types, but it comes with challenges, according to Giacomo Vacca, president and founder of Kinetic River (Mountain View, CA). The spectral emission overlap between the various markers causes many problems, from a limit to the maximum number of markers measurable in any given sample to a high burden on operators and high costs of reagents and controls to perform the compensation procedures typically required using purely spectral methods.
To address the existing challenges, Vacca’s team developed and patented time-resolved flow cytometry (TRFC), making the entire cell-analysis process faster, easier, more efficient, and more powerful.
TRFC works by delivering modulated bursts of light to cells in a flowing sample (instead of using continuous-wave excitation). The rapid modulation of the excitation light, combined with fast detection electronics, allows the collection of detailed nanosecond-scale information on fluorescence decays. Kinetic River’s proprietary algorithms use this information to distinguish emissions from different fluorophores, even when their spectral emission curves overlap completely or are essentially identical cases where spectral unmixing struggles or fails entirely (see Fig. 2).Analyzer tools
Further advancement of TRFC is evident in the development of two new analyzer tools: Arno and Colorado.
Arno technology provides compensation-free flow cytometry. With a Phase II SBIR grant from the National Institutes of Health (NIH), Kinetic River has developed an analyzer to perform compensation-free 12-marker flow cytometry assays with just two lasers and six detectors using the time-resolved methods.
By discriminating fluorescent markers by their color as well as by the decay of their fluorescence emissions (a.k.a., their lifetime), Arno avoids several issues that hamper existing flow cytometers, explains Vacca.
“Eliminating the need for spectral compensation means users won’t have to spend time measuring and compensating for spectral spillover,” he says. “And they won’t have to spend money on the controls and reagents currently needed to perform compensation calibration. The quality of results will also increase, since compensation introduces greater uncertainty in the measurements—something called spreading of cell population clusters in flow cytometry.”
Arno uses TRFC to assign two fluorophores to each physical detector—stacking their lifetimes and subsequently doubling the number of measurable parameters for a given number of lasers and detectors.
“This stacking, in turn, makes it possible to achieve a desired number of parameters without cramming fluorescence emissions in the available spectral window, keeping them well separated from each other,” he says. In conventional analyzers, cramming causes crosstalk that forces compensation (unmixing), a practice that involves gathering crosstalk measurements via costly reagents from all channels into all other channels and creating a mathematical inversion matrix that compensates for such crosstalk.
Vacca’s team demonstrated doubling the number of markers measurable on each detector, paving the way to tripling them. Lifetime-based discrimination allows emissions from multiple markers to be collected by each detector, greatly expanding the analytical power of flow cytometry while minimizing the number of lasers and detectors needed in a system (see Fig. 4).The TRFC-based Colorado analyzer tool, also supported by a NIH Phase II SBIR grant, enables autofluorescence-free flow cytometry. This tool is designed as a general-purpose cell analyzer with automated autofluorescence removal. It can handle up to 16 colors or fluorescent markers, automatically identifying and subtracting the autofluorescence background from the detected signals in selected channels (where autofluorescence is a problem). Existing efforts to handle autofluorescence can impair sensitivity or make certain detection channels unusable altogether.
“Certain cell types have a particularly strong autofluorescence,” he says, “which obscures the fluorescence from externally added (i.e., exogenous) fluorophores, reducing the sensitivity of the measurement and limiting the usefulness of certain assays. By identifying and automatically subtracting the autofluorescence background based on its different fluorescence lifetime from desired emission signals due to exogenous fluors, the Colorado increases instrument sensitivity and an assay’s resolution of negative and positive populations.”
More specifically, it can distinguish an autofluorescence background from the fluorescent signals of added cell markers, even when those emissions have completely overlapping spectra, resulting in a radical increase of sensitivity of flow assays for certain cell types.
Kinetic River is now advancing toward translating their work to the challenging task of efficiently discriminating cancer and normal cells in flow cytometry.
Applications for TRFC
In addition to compensation-free flow cytometry and autofluorescence-free flow cytometry, applications unleashed by TRFC include rapid, label-free cancer screening. One specific example with enormous practical potential leverages the autofluorescence emissions of ubiquitous metabolic compounds NADH and FAD present in all cells. The autofluorescence of these molecules exhibits different lifetimes depending on whether the molecules are free or enzyme-bound.
“By measuring the proportion of autofluorescence emissions from the free and bound fractions of these compounds, one can establish a metabolic signature of each cell, he says. “This has been exploited in fluorescence lifetime microscopy to distinguish, for example, normal cells from cancerous ones based solely on autofluorescence, since cancer cells have anomalous metabolic signatures.”
Vacca has begun development of a special-purpose analyzer, the Tiber, to examine blood samples and disaggregated tissue samples. This could allow effective, label-free detection and instant assessment of cancer cells. “The advantages would be huge, starting with the rapidity of delivering such results to the doctor and to the patient,” he says.
Another potential application for TRFC is screening for circulating tumor cells (CTCs) in the bloodstream. Existing commercial technology for this purpose is based on magnetic capture targeting a surface antigen (Epcam) expressed by a number of cancer cells—but this technique is subject to well-known limitations, such as lengthy processing and completely missing cancer cells that don’t express that antigen. By skipping the added step of labeling cells with external markers and going to direct measurements of compounds already in the cells, according to Vacca, TRFC could deliver a much less expensive, faster, and more sensitive CTC analysis than is currently possible.
Looking toward the future
“We envision TRFC technology to provide flow cytometry users with capabilities they would not otherwise have,” Vacca says.
All flow cytometry systems currently used must perform compensation, which poses a significant labor and cost burden on core labs. “By delivering an instrument where compensation is a thing of the past, users will be able to spend more time on value-added activities like carefully analyzing their data instead of calibration,” says Vacca. And, “autofluorescence-free flow cytometry will lift a veil from the results users currently see, making it possible to push their assay sensitivity to levels previously unattainable.”
Label-free rapid cancer screening is also on the horizon. With this technique, autofluorescence is the signal of interest instead of the background to be subtracted. By measuring autofluorescence from unlabeled cells, TRFC could distinguish between normal and cancerous cells without the need for fluorescent markers.
The hope is to expand the number of detection channels drastically. With TRFC, each physical detector can measure multiple markers. Vacca’s team has already demonstrated doubling of the total number of markers detectable with each sensor. “And we’re on a path to tripling them,” he notes. “This will open up a space where assays with 40+ colors can be performed—and eventually even coupled with cell sorting.”
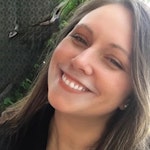
Justine Murphy | Multimedia Director, Digital Infrastructure
Justine Murphy is the multimedia director for Endeavor Business Media's Digital Infrastructure Group. She is a multiple award-winning writer and editor with more 20 years of experience in newspaper publishing as well as public relations, marketing, and communications. For nearly 10 years, she has covered all facets of the optics and photonics industry as an editor, writer, web news anchor, and podcast host for an internationally reaching magazine publishing company. Her work has earned accolades from the New England Press Association as well as the SIIA/Jesse H. Neal Awards. She received a B.A. from the Massachusetts College of Liberal Arts.