PHOTONIC FRONTIERS: THE EXTREME LIGHT INFRASTRUCTURE: The ELI aims to break down the vacuum
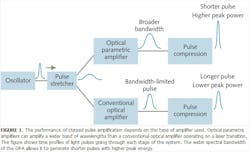
The Extreme Light Infrastructure (ELI) is a big, bold European plan to increase the peak power from ultrashort-pulse lasers by two orders of magnitude to 200 PW. Focusing those pulses in time and space should produce peak intensities of 1025 W/cm2, says project coordinator Gerard Mourou, director of the Institut de Lumière Extrême (Institute for Extreme Light; Palaiseau, France). Mourou's goal is to explore new frontiers in physics, including trying to trigger the breakdown of the vacuum, the fabric of space-time itself.
This year marks the start of the delivery phase after a three-year preliminary phase of planning. The European Commission will spend €280 million (US$370 million) on each of the first three "pillars" of the infrastructure—dedicated ultrafast laser laboratories in the Czech Republic, Hungary, and Romania. The project will help the three eastern European nations develop their own scientific infrastructure while building new labs around 10-PW-class lasers. The projects will also lay the groundwork for building the 200 PW laser at a site to be selected in 2012.
Technical foundation
Mourou and his student Donna T. Strickland—just elected vice president of the OSA—laid the cornerstone for ELI when they invented chirped-pulse amplification in the mid-1980s at the University of Rochester. (Rochester, NY). Their trick is to pass ultrafast pulses through a dispersive medium before amplifying them. This chirping process spreads the wavelengths in the pulse over a much longer interval, reducing the peak power before amplification. Reducing peak power reduces the chance of optical damage to the amplifier. After amplification, a final pulse compressor reverses the dispersion process, squeezing the amplified light in time to recreate the ultrashort pulse, this time with much higher amplitude.
Chirped pulse amplification (CPA) led to dramatic increases in the peak power of short pulses, but the process is limited by the gain bandwidth of Ti:sapphire amplifiers, which restricts minimum pulse duration. Broader bandwidth and shorter output pulses are possible using optical parametric chirped pulse amplification (OPCPA)—in which an optical parametric amplifier replaces the Ti:sapphire amplifier—because the nonlinear process is not limited to the gain band of a laser transition (see Fig. 1).
The final design of the ELI may use either an OPCPA or a hybrid with a final Ti:sapphire chirped-pulse amplifier. "You want to boost the pulse power to a certain level with the broadest bandwidth possible," says Mourou. For example, an OPCPA could amplify nanojoule pulses by a factor of 106 to 107, then the final stage could be a CPA, which would not reduce bandwidth dramatically because it provides only part of the amplification. "CPA gives you the energy, and OPCPA gives you the bandwidth," says Mourou.
First step to 10 PW
The 10 PW Apollon laser being built at the Institute for Extreme Light is a crucial step in developing ELI. This single-beam hybrid system starts with a high-repetition rate femtosecond Ti:sapphire oscillator delivering 100 mJ pulses to an OPCPA powered by diode-pumped thin-disk ytterbium lasers at 100 Hz. Output of that stage will be amplified in large Ti:sapphire lasers generating at least one pulse per minute, then fed through a pulse-compression grating.Challenges in taking that big step beyond today's petawatt lasers include developing powerful doubled-neodymium pumps for Ti:sapphire amplifiers, avoiding optical damage to the amplifiers, and developing large broadband compression gratings that can withstand the high laser flux. Plans call for Apollon to deliver 5–7 PW pulses by the end of 2013, and ultimately to deliver 150 J in a 15 fs pulse, for a peak power of 10 PW in 2015. That should be sufficient to reach power densities of 1023 to 1024 W/cm2.
Mourou says the three new infrastructure pillars will be based on the Apollon design to pursue different ranges of petawatt applications. Hungary will focus on attosecond physics, the Czech lab on beams of radiation produced by intense laser-matter interactions and laser acceleration, and the Romanian lab will focus on nuclear interactions (see Fig. 2).
Three variations on a petawatt theme
The Hungarian ELI seeks to expand the frontiers of attosecond science by using the relativistic Doppler effect. Lower-power femtosecond pulses can produce high-order harmonic pulses as short as about 100 as by exciting electrons in suitable gases. With peak power in the 10 PW range, the Hungarian ELI will accelerate electrons in a laser-produced plasma to relativistic speeds in femtoseconds, and those relativistic electrons can Doppler-shift incident photons. One idea is to focus a 10 PW pulse onto a thin-foil target, so the intense fields accelerate electrons coherently to form a moving mirror that Doppler-shifts the laser light to much shorter wavelengths and compresses the pulse duration. Developers estimate this approach should be able to generate 10 as pulses.
"Driving and controlling matter in such extreme conditions, to obtain a new generation of attosecond light sources, is a rich and challenging scientific project in its own right," says a report on the "grand challenges" of ELI. Key issues include producing laser pulses that can control plasma interactions on the required time scale and developing measurement techniques suitable for such short pulses.
The Czech pillar of ELI will focus on using high-intensity laser fields to produce beams of high-energy particles and radiation in the x-ray and gamma-ray ranges. The concepts have been demonstrated at lower laser intensities, but ELI's much higher intensity and the high repetition rate for the Czech pillar promise important advances in pulse duration and brightness.
At ultrahigh laser intensities, nonlinear effects shift laser acceleration of charged particles into a "bubble regime," in which the wake field present at lower field intensities takes the form of a solitary bubble. This can efficiently accelerate large numbers of electrons to relativistic speeds and trap them in dense bunches with near-uniform energy. Electron pulses are synchronized with the laser pulses, as needed for pump-probe experiments. Models show that in its early stage ELI should be able to generate ultrashort bursts of gigaelectronvolt (GeV) electrons, an important step toward developing compact laser plasma accelerators.
Scaling to 10 PW should generate even higher-energy electrons, and ELI could be used in experiments to evaluate issues in scaling electron accelerators to the teraelectronvolt (TeV) range. Another possibility is focusing a second intense femtosecond laser pulse onto the laser-generated electron pulse, to produce intense x-ray or gamma-ray pulses by Compton scattering.
More practical applications also are possible. Compact petawatt lasers with high repetition rates could accelerate protons to the 70 to 250 MeV energies needed for cancer therapy. That would be an important advance in size, cost, and usability over the massive cyclotrons needed to accelerate protons to that energy.
The Romanian pillar of ELI, near Bucharest, will probe the atomic nucleus with photons—photonuclear physics. Because nuclear energies are orders of magnitude higher than those of individual optical photons, these experiments will require very high laser beam intensities, generating very high energy photons, or both.
To allow a broad range of experimental possibilities, the facility will include an electron accelerator as well as three 10 PW laser beam lines like Apollon. With the goal of reaching laser intensities near 1024 W/cm2, the system will operate at modest repetition rates of 0.1 to 1 Hz. One possibility is studying effects of illuminating nuclei with such high intensities of optical photons. A second is generating tunable pulses of kilovolt to multi-MeV photons by Thomson scattering of the intense optical pulses off bunches of high-energy electrons. Although much planned research is fundamental, studies of photonuclear physics also might lead to innovations in nuclear waste disposal.The ultimate ELI
The first three pillars of ELI should yield exciting results by themselves, but they also will serve as stepping stones for an even more extreme and challenging infrastructure—a 200 PW laser able to deliver intensities of 1025 W/cm2 (see Fig. 3).
The technology has yet to be finalized, and the site will not be chosen until 2012, but Mourou has bold plans. He envisions coherently adding the outputs of about 10 Apollon-class lasers. The beams will converge from around a sphere to focus on the smallest possible point to achieve the highest possible intensity. The most exciting results may come from focusing at nothing. Quantum electrodynamics predicts that at extremely high field intensities, the vacuum will break down, forming electron-positron pairs. Theoretical predictions of the threshold for breakdown vary widely, as do those of near-threshold effects.
If all goes well the ultrahigh-intensity fourth pillar of ELI should come on-line in 2017 and try to go where no laser has gone before—to break down the vacuum. But even if that goal is beyond reach, the Extreme Light Infrastructure should produce some extremely important knowledge.
FURTHER READING
1. Extreme Light Infrastructure web site, at http://www.extreme-light-infrastructure.eu
2. Extreme Light Infrastructure: Report on the Grand Challenges Meeting (European Commission, December 2009), available at http://www.extreme-light-infrastructure.eu/pictures/Grand-Challenges-Meeting-Report-id66.pdf
3. F. Amiranoff et al., Proposal for a European Extreme Light Infrastructure (ELI), available at http://www.extreme-light-infrastructure.eu/pictures/ELI-scientific-case-id17.pdf
4. T. Tajima, Scientific Advisory Committee of Extreme Light Infrastructure: Report on the ELI Science (May 2009), available at http://www.extreme-light-infrastructure.eu/pictures/Scientific-Advisory-Committee-SAC-Report-id65.pdf
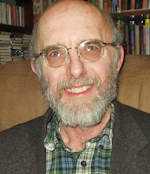
Jeff Hecht | Contributing Editor
Jeff Hecht is a regular contributing editor to Laser Focus World and has been covering the laser industry for 35 years. A prolific book author, Jeff's published works include “Understanding Fiber Optics,” “Understanding Lasers,” “The Laser Guidebook,” and “Beam Weapons: The Next Arms Race.” He also has written books on the histories of lasers and fiber optics, including “City of Light: The Story of Fiber Optics,” and “Beam: The Race to Make the Laser.” Find out more at jeffhecht.com.