Photonic Frontiers: Laser diodes: Looking back/Looking forward: Laser diodes have come a long way and brought five Nobel prizes
The observation of incredibly bright recombination radiation from gallium-arsenide diodes cooled to liquid-nitrogen temperature at the MIT Lincoln Laboratory (Lexington, MA) launched the laser diode. The light was so bright that one person who heard Bob Keyes' talk at the July 1962 Solid State Device Research Conference thought it violated the laws of thermodynamics. Keyes said, "I'm sorry," but it was no mistake. By the end of 1962, four independent teams inspired by it had made the first laser diodes, which were pulsed at cryogenic temperatures.1 Figure 1 shows researchers at the General Electric Research & Development Center (Schenectady, NY) where they made the first laser diode in 1962.
The next year brought a crucial concept that would shape the future of laser diodes and earn the 2000 Nobel Prize in Physics for its two independent inventors half a world apart. Herbert Kroemer, then at Varian Associates in California, could not get funding to follow up on his idea. However, Zhores Alferov went on to make the first heterojunctions with Rudolf Kazarinov at the Ioffe Physico-Technical Institute (St. Petersburg, Russia). By controlling the flow of current and light, the heterostructure has been crucial to the success of laser diodes.The first issue of Laser Focus on January 1, 1965, contained two laser diode stories. An IBM team used a 12-oz. "music pistol" to send low-bandwidth audio across a room with pulses from a GaAs laser (see Fig. 2). A separate IBM group claimed 1 W of continuous output from an 840 to 850 nm GaAs laser cooled to the 77K temperature of liquid nitrogen.
The May 1, 1965 issue on p. 13 cited a talk by Bob Rediker of the Lincoln Lab team, who predicted it would be possible to make a laser diode "flashlight" with continuous-wave output of 100 W at room temperature. However, his lab's best was a cryogenically cooled 1.5 W laser diode.
One paper in the April 1, 1965 issue was far ahead of its time. Ivars Melngailis of Lincoln Lab made the first "longitudinal injection laser" (see Fig. 3). Light oscillated between the polished top and bottom surfaces of an indium antimonide diode, emitting 50 ns pulses at 5.2 microns parallel to current flow. Its threshold current was 20 A when operated at 10K, and it was 220 μm thick, so it was far from practical, but it presages the modern vertical cavity surface-emitting laser (VCSEL).2Laser diodes also made their first trip into space in 1965. The August 1 issue described plans for Gemini 7 astronauts Frank Borman and James Lovell to test a voice downlink to ground using four laser diodes emitting a total of 16 W at 900 nm. They were to aim the downlink at green argon beams from ground stations. NASA paid RCA $88,657 to build three 6-pound diode transmitters. But the December experiment was hindered by bad weather, and their signals were not spotted on the ground.3
CW operation at room temperature remained elusive until heterojunctions could be deposited both above and below the active layer. Alferov’s group succeeded with a stripe-geometry laser in the spring of 1970, just weeks ahead of Mort Panish and Izuo Hayashi at Bell Labs with a broad-area diode. However, with the Iron Curtain hindering communication, we and Bell Labs thought they were first, and we credited them in the October 1970 issue. Bell Labs officials downplayed prospects for immediate applications. The company’s head of transmission research, Rudy Kompfner, did not expect laser diodes to be used in communications systems for more than a decade, and other system specialists put them at 20 years away. All were keenly aware that laser diodes would have to last far longer than the couple of hours demonstrated in the lab.
It took five years for CW room-temperature laser diodes to reach the market, and when they did, I wrote the new product story (see Fig. 4). A small New Jersey company called Laser Diode Laboratories offered 5 or 10 mW models at 800, 850 or 900 nm for $250 to $350 each. Bell Labs had reported such lifetimes a year earlier, and Laser Diode’s life tests had only reached 1000 hours. However, they were confident enough to sell the lasers, packaged in standard transistor-type cans.By the spring of 1976, Bell was extrapolating average room-temperature lifetimes of 5 to 10 years for laser diodes. After a further year of accelerating aging tests, we reported in August 1977 that Bell projected million-hour diode lifetimes—good enough for the telephone network. Their remarkable improvements in GaAs laser diode technology were a tour de force of what Bell Labs engineers could achieve.
Longer and shorter wavelengths
Ironically, that success came just as fiber system development was shifting to longer wavelengths where fiber loss was lower. That required new semiconductor materials, and our February 1979 issue reported rapid progress on new InGaAsP compounds. J. J. Hsieh of Lincoln Lab had made CW single-mode InGaAsP lasers that emitted several milliwatts at 1300 nm for 1700 hours.4 A team at the Tokyo Institute of Technology had made distributed Bragg reflector InGaAsP diodes. Those were impressive feats given that Hsieh had just reported the first 1250 nm InGaAsP laser in April 1977. Our May 1979 issue described commercial single-mode InGaAsP lasers emitting 3 mW at 1300, introduced by Mitsubishi Electric for $2390 to $2590. It was the start of a shift in the communications market.
Yet a big new market began to open for GaAlAs lasers when Philips NV (Eindhoven, Netherlands) demonstrated a preliminary version of its audio Compact Disc player, based on a 780 nm GaAlAs laser, described in our July 1979 issue (see Fig. 5). Helium-neon lasers had been used in videodisk players, but Philips considered diodes essential for audio players. The first CD players sold for about $1000 each in 1982, but as prices dropped, billions would be sold.Our September 1979 issue reported another new use for GaAlAs lasers: smaller versions of the laser computer printers originally developed around gas lasers. Canon USA (Lake Success, NY) said it would introduce an OEM desktop printer based on an 810 nm GaAlAs diode priced at $12,000 to $15,000. The market grew slowly until Apple introduced its LaserWriter printer in 1985 for $6995. The latest one I bought cost under $100 a couple of years ago.
The first room-temperature visible laser diodes emerged in 1985 when Sony demonstrated lasing at 671 nm from AlGaInP. Two years later, Tohru Suzuki of NEC highlighted his report to CLEO 1987 on operation of 678 nm GaInP diodes for more than 4500 hours at room temperature by wielding a red diode pointer. Soon they were standard presenter tools, and they later found uses from DVD players to cat toys.
Another 1985 first was room-temperature operation of VCSELs by Kenichi Iga of the Tokyo Institute of Technology, who conceived of the idea in 1977, independent of Melngailis's earlier work. Later Larry Coldren of the University of California at Santa Barbara added deposition of multilayer DBR mirrors on top and bottom during device fabrication. VCSELs have replaced edge-emitting diodes for many low-power applications because they can be made and packaged inexpensively and offer better beam quality.
High-power diodes and diode pumping
CLEO 1987 also saw a rather different laser-diode trend: high powers to pump solid-state lasers, as we reported in our July 1987 issue. Laser diode power had risen steadily over the years, aided by the integration of many laser stripes on a single semiconductor bar, pioneered in 1978 by Don Scifres and colleagues at the Xerox Palo Alto Research Center, who generated more than 100 mW per facet.5
GaAlAs was the right stuff for diode pumping because it could efficiently generate light in the 808-nm pump band of neodymium lasers. Scifres went on to commercialize the technology at Spectra Diode Labs (San Jose, CA), which showed high-power diodes including a 1 W quantum-well phased array emitting at 800-840 nm at CLEO 1987. Other companies showed diode-pumped lasers, including Spectra-Physics (Mountain View, CA), Lightwave Electronics (Mountain View), A-B Lasers (Concord, MA), and Amoco Laser (Naperville, IL). Spectra-Physics featured three diode-pumped lasers on its back-cover ad in July 1987 (see Fig. 6), emitting CW or Q-switched at 1.06 μm or pulsed at the green second harmonic.The surprising blue laser diode
A series of refinements in diode structure and fabrication brought the advances of the 1980s. The blue laser diode emerged from surprising breakthroughs that earned the three men behind them the 2014 Nobel Prize in Physics.
I got a preview at a Materials Research Society meeting in December 1991, when a friend said "you've got to see this," and introduced me to Isamu Akasaki from Nagoya University. He pulled from his pocket what looked like a red laser pointer, but it emitted bright blue light. Akasaki explained how he and Hiroshi Amano had reportedly made the first bright blue LEDs by taming gallium nitride, which previously had been limited to very inefficient operation. In 1993, Shuji Nakamura scored a second breakthrough at Nichia Chemical Corp. (Tokushima, Japan), leading to 2.7% conversion efficiency and bright blue commercial LEDs.6
In an August 1994 feature on prospects for blue lasers, two SDL researchers noted the blue GaN LEDs, but wrote "commercial semiconductor lasers based on these materials are at least several years away," and recommended harmonic generation from near-infrared diodes. Other researchers continued development of ZnSe laser diodes, and our December 1994 reported a record 463 nm.
But that didn't deter Nakamura. In November 1995, he wowed the Materials Research Society by showing an array of bright blue and green LEDs, then went home and demonstrated the first InGaAs laser diode, emitting pulses at 417 nm.7 "The GaN materials could provide tough competition for II-VI lasers," I wrote in our March 1996 issue.
That would prove an understatement. GaN diodes blew away short-lived II-VI lasers. It was the answer to the dreams of optical disk developers, who wanted 405 nm violet light to squeeze a full HD movie onto a 12 cm disk. A format war and downloads shrank the market for Blu-Ray, but bright blue GaN LEDs based on the same technology proved a boon for solid-state lighting.
Higher sales and higher powers
The telecommunications market boomed with the growth of the Internet in the mid-1990s, and our January 1997 market review estimated sales of telecommunication lasers accounted for more than half of the $1.6 billion global market for laser diodes in 1996. Laser diode sales, in turn, were more than half of the total $2.8 billion market for all lasers in 1996.
High-power diode sales were much lower, but growing fast. In our December 1994 technology review, we noted they had gone beyond diode pumping to make inroads in graphic arts, medicine, and materials-working. Linear GaAlAs arrays had reached CW powers of 70 W, and those bars could be stacked in two-dimensional arrays emitting peak power to 5 kW in quasi-CW operation. New growth techniques enabled InGaAs diodes to deliver high powers in the 900 to 1000 nm band for pumping erbium and ytterbium and for direct-diode applications.
The first direct-diode applications were soldering and plastic welding. Mercedes Benz used direct diodes to weld plastic for its first remote-control car key, recalls Georg Treusch, GM of TRUMPF Photonics (Farmington, CT). That system coupled 40 to 60 W from bars into the round core of a beam-delivery fiber. Now direct diodes can deliver multikilowatt powers. "Braising and welding need 4 to 6 kW and require spot sizes of 400 to 600 μm, which direct diodes can deliver," says Treusch.
Cutting metals requires higher intensity and spot sizes of only 100 μm, and reaching those levels with diodes requires beam combination, as we described in the June 2012 issue. TeraDiode (Littleton, MA) now can deliver 2 kW through a 100 μm fiber by multiplexing many wavelengths. The big question is how the cost will compare to a disk or fiber laser, says Treusch. However, he notes that both fiber and thin-disk lasers require laser diode pumps. Diode pumping now goes all the way to the top in solid-state laser power, driving both fiber and slab lasers that can deliver CW 100 kW beams.
Our January 2015 market review put laser diode sales at 44% of the $9.4 billion global laser market in 2014—but that does not include pump diodes built into fiber or solid-state lasers. We did not calculate what fraction of diode-pumped laser sales can be attributed to built-in pump diodes, but it clearly is substantial. So if we were able to add the value of those pump diodes to that of other laser diodes, it would bring their share of the laser market to more than half.
Looking forward
Laser diodes have a bright future in materials working, says Treusch. "Dense wavelength division multiplexing can couple single-mode emitters over a wide range of wavelengths to achieve single-mode output in the multikilowatt range." But he expects diodes to continue sharing the market with fiber, thin-disk, and carbon-dioxide lasers, with each finding its own place because application requirements vary widely. For example, engineers prefer flexible fiber beam delivery for heat treating because it covers the complex geometry of automotive parts better than wide beams from direct-diode arrays.
Peak diode efficiency is now 72 to 74%, although it drops at higher operating power. "Everybody is working on incremental improvements," says Treusch, and it may be possible to reach 80%. Those levels can't be matched by any other electrically excited laser.At the other end of the size and power scale, nanolasers have become a hot research field. Surface plasmon effects at the boundary between a semiconductor and a metal can concentrate energy into a volume smaller than a cubic wavelength, as I wrote in "Nanolaser Progress: Surface plasmons and nanolasers" in the September 2012 issue (see Fig. 7). The small volumes promise fast modulation and low threshold, but concentrating the emission is a challenge. Nano-scale LEDs might make better light sources, suggests Jacob Khurgin of Johns Hopkins University (Baltimore, MD).8 However, that raises the question of what's the difference between a laser and an LED on that scale.
Semiconductor materials are another frontier. Our senior editor John Wallace wrote in May 2014 that green InGaAs laser diodes have reached the market (see Fig. 8). However, commercial InGaAs lasers have yet to reach wavelengths beyond 530 nm, leaving a laser-diode void in the yellow, as well as ultraviolet wavelengths shorter than 370 nm. LEDs may fit some of these niches.With most laser diodes hidden inside larger systems, it's easy to miss the extent of their impact, which goes far beyond five Nobel prizes. Without laser diodes, "there would be no Internet," said David Welch, cofounder and president of Infinera (Sunnyvale, CA), speaking at the Laser & Photonics Marketplace Seminar on the 50th anniversary of the laser diode.
References
1. J. Hecht, "The breakthrough birth of the laser diode," Opt. & Photon. News 38 (July/Aug 2007).
2. I. Melngailis, "Longitudinal injection-plasma laser of InSb," Appl. Phys. Lett. 6, 59–60 (1965).
3. Gemini 7: The NASA Mission Reports.
4. J. H. Hsieh and C. C. Shen, "Room-temperature CW operation of buried-stripe double-heterostructure GaInAsP/InP laser diodes," Appl. Phys. Lett. 30(8), 429–431 (1977).
5. D. R. Scifres, R. D. Burnham, and W. Streifer, "Phase-locked semiconductor laser array," Appl. Phys. Lett. 33(12), 1015–1017, 1978.
6. S. Nakamura, T. Mukai, and M. Senoh, "Candela‐class high‐brightness InGaN/AlGaN double‐heterostructure blue‐light‐emitting diodes," Appl. Phys. Lett. 64, 1687 (1994).
7. S. Nakamura et al., Jpn J.Appl. Phys. 35, L74–L76 (Jan. 15, 1996).
8. J. B. Khurgin and G. Sun, "How small can 'Nano' be in a 'Nanolaser'?" Nanophotonic 1, 3–8 (2012); DOI: 10.1515/nanoph-2012-0017.
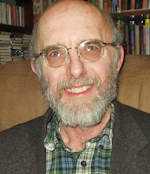
Jeff Hecht | Contributing Editor
Jeff Hecht is a regular contributing editor to Laser Focus World and has been covering the laser industry for 35 years. A prolific book author, Jeff's published works include “Understanding Fiber Optics,” “Understanding Lasers,” “The Laser Guidebook,” and “Beam Weapons: The Next Arms Race.” He also has written books on the histories of lasers and fiber optics, including “City of Light: The Story of Fiber Optics,” and “Beam: The Race to Make the Laser.” Find out more at jeffhecht.com.