OPTICALLY PUMPED SEMICONDUCTOR LASERS: Green OPSLs poised to enter scientific pump-laser market
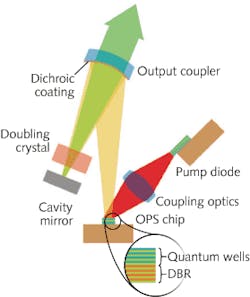
Optically pumped semiconductor lasers (OPSLs) have been optimized for an increasing number of applications based on continuous-wave (CW) visible and, most recently, UV laser light. Specific applications include bioinstrumentation at 488 and 355 nm with less than 0.5 W output power, multiwatt portable forensic and lightshow systems at 460, 532, and 577 nm, and multiwatt 532 and 577 nm lasers for ophthalmology. All these applications have benefited from OPSL technology because of features that include wavelength flexibility and power scalability, among others, in a compact package.
In terms of power, mode quality, and noise requirements, one of the most demanding applications for CW visible lasers is pumping Ti:sapphire ultrafast systems. Such systems enable cutting-edge research across many disciplines ranging from terahertz imaging to time-resolved spectroscopy. The recent release of an ultralow-noise OPSL providing up to 5 W of stable output at 532 nm means these ultrafast systems can now be pumped by OPSLs.
OPSL basics
The gain medium of an OPSL is a monolithic III-V semiconductor chip containing layers of tertiary indium gallium arsenide (InGaAs) quantum wells alternated between binary gallium arsenide (GaAs) layers. These binary layers are optimized to efficiently absorb pump radiation, resulting in a high population of charge carriers. This leads to population inversion and recombination in the quantum wells, which emit at a wavelength determined by the stoichiometry and physical dimensions of the quantum-well structures. Behind these absorption/emission layers, the gain chip also incorporates several alternating high-index and low-index layers that act as a low-loss distributed Bragg reflector (DBR) optimized for maximum reflectivity at the specific OPSL output wavelength, and which forms one of the cavity mirrors.Near-infrared (IR) pump light is provided by a diode array. In the standard configuration used by Coherent in these OPSLs, the pump output is focused on the front surface of the semiconductor chip using conventional lenses (see Fig. 1).
This proven OPSL format is optimal for several reasons. Similar to vertical-cavity lasers (VCSELs) and unlike edge-emitting devices, the OPSL chip emits light perpendicular to the diode junction. This vertical emission is one of the keys to the excellent spatial mode performance of an OPSL, particularly at higher powers, which requires a larger-area chip. However in the case of a VCSEL there is no way to flood a large-area emitter with charge carriers without using extended electrodes that would introduce excessive loss. Optical pumping overcomes this limitation in the OPSL. Furthermore, OPSL technology allows the use of external optics to design advanced laser cavities and tailor output beam characteristics to the intended application. Access to the intracavity beam facilitates efficient and simple doubling of the near-IR output to produce a visible wavelength, or even frequency tripling to obtain UV output. In addition, multiple OPSL chips can be used inside the same cavity to effect power scaling (see Fig. 2).
Wavelength flexibility
One of the main advantages of OPSLs is their wavelength flexibility. The fundamental near-IR output wavelength is determined by the structure of the InGaAs gain chip, and can be set anywhere from 920 to 1154 nm, yielding frequency-doubled output between 460 and 577 nm. This flexibility has enabled a paradigm shift in the way laser applications are supported. Historically, design engineers have had to choose from available laser sources for a wavelength that most closely met the needs of the application. With an OPSL, however, the laser wavelength can be tailored to exactly match the application. An example of this is the 3 W 577 nm laser designed for ophthalmology that won the PhAST/Laser Focus World Innovation Award in 2008. The 577 nm wavelength was chosen to coincide with a strong absorption peak of oxy-hemoglobin, enabling superior photocoagulation results with better tissue discrimination for sufferers of wet-form age-related macular degeneration (AMD).
Another advantage of OPSLs over traditional diode-pumped (DPSS) lasers is efficient and simple pumping of the semiconductor gain medium. Specifically, the pump wavelength needs only to be shorter than the bandgap of the GaAs. So there is no need to select pump diodes based on their precise wavelength characteristics, which reduces yield, and a feedback temperature-control loop to stabilize the pump wavelength is unnecessary.
Partly because of these relaxed design tolerances, OPSLs have proved to be extremely reliable. With more than 20,000 installed units, lifetime data shows that the OPSL is the most reliable laser technology ever produced by Coherent. The higher reliability translates into a marked reduction in cost of ownership compared to other CW laser technologies, including ion and DPSS lasers.
Power scaling
Pumping Ti:sapphire lasers and other scientific applications required developing an OPSL product with adequate power (multiple watts) and low noise. With the recent launch of the Coherent Verdi G-Series, it is useful to review the characteristics of OPSL technology that enabled these performance goals to be met.
Like its DPSS predecessors, an OPSL generates near-IR fundamental output that must then be frequency-doubled using an intracavity nonlinear crystal. The OPSL is capable of producing hundreds of watts of IR intracavity power allowing very efficient nonlinear conversion to green at multiwatt levels. The OPSL architecture offers several options for power scaling. These include increasing the area of the gain chip, increasing the pump power, and even using multiple gain chips in series. We have shown in the laboratory that combining all these methods can take OPSL output beyond 70 W.
Increasing the output power in any solid-state laser raises the thermal load in the gain medium, but because the OPSL gain medium is a thin semiconductor wafer efficiently cooled from its rear submount, it is possible to operate the chip at multiwatt output levels. More important, it produces virtually no radial thermal gradient, so there is no thermal lensing. In contrast, solid-state lasers based on a diode-pumped, rod-shaped gain medium all produce thermal-lens effects that must be exactly balanced by other cavity optics. This is the reason that many commercial solid-state lasers can only produce their target beam characteristics over a narrow operational power range.
Harmonic generation
Two aspects of frequency doubling in OPSLs merit closer examination. In Nd:YAG or vanadate lasers with intracavity doubling and multiple longitudinal modes, the frequency-doubled output displays significant intensity noise due to second-harmonic (doubling the frequency of one longitudinal mode) and sum-frequency generation (adding the frequencies of two different longitudinal modes). Sum-frequency generation couples individual longitudinal modes and thereby enables direct dynamic interactions between longitudinal modes. As power distribution between the modes change, the intensity of one mode depends on the gain of another, which generates significant intensity noise, called "green noise."1In some commercial lasers, a longer (and potentially less stable) cavity having a high density of longitudinal modes is used in an attempt to reduce the overall green noise. Specifically, with a larger number of modes within the effective gain bandwidth, the noise from all the pair-wise interactions somewhat averages out. The most effective and elegant remedy is to confine the laser performance to a single longitudinal mode, which completely eliminates green noise and provides the additional benefit of long coherence lengths.
Unlike multi-longitudinal-mode Nd-based lasers, OPSLs do not exhibit green noise and deliver better noise performance than diode-pumped solid-state (DPSS) lasers, but without the need for line-narrowing optics, stabilization loops, and precise control over cavity temperature. The fundamental difference in behavior results from the short excited-state lifetime of the OPSL gain medium. With no stored gain, dynamic fluctuations found in DPSS lasers are completely absent in an OPSL and the longitudinal mode-pattern is thus entirely determined by the characteristics of the laser cavity. With this completely static mode pattern, there are no fluctuations in the overall doubling efficiency, and therefore no "green noise." Hence the latest 5 W OPSL (Coherent Verdi G5) delivers rms noise performance of better than 0.03% over a bandwidth of 10Hz to 100MHz, making it ideal for ultra-low-noise applications in Ti:sapphire pumping (see Fig. 3).
Intracavity second-harmonic generation (SHG) in an OPSL is not only a low-noise process, but also a very efficient one. This is because SHG scales with the square of the circulating power in the near-IR fundamental, which is markedly higher in an OPSL than in a DPSS with the same diode pump intensity. The InGaAs is a relatively low gain material so the cavity supports a high-saturation power level and the circulating power vs. diode current stays linear to higher power levels.
REFERENCES
1. T. Baer and references therein, J. Opt. Soc. Am. B: Opt. Phys 3(9) p. 1175 (Sept. 01, 1986).
Marco Arrigoni
Marco Arrigoni is vice president of marketing at Light Conversion (Vilnius, Lithuania). He previously served as director of marketing at Coherent (Santa Clara, CA) from 2007 through 2023.
Arnaud Lepert | General Manager, HOPS Business Unit at Coherent
Arnaud Lepert is General Manager, HOPS Business Unit at Coherent (Santa Clara, CA).
Brandon Morioka | Product Manager, Coherent
Brandon Morioka is a Product Manager at Coherent (Santa Clara, CA).