Multiphoton Imaging/Neuroscience: Ultrafast lasers take neuroscience deeper yet
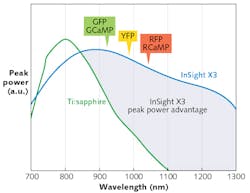
JULIEN KLEIN
Neuroscience continues to further our understanding of the brain both structurally and functionally. Technological developments are enabling observation of larger neural networks deeper in the brain with improved sensitivity and selectivity, and facilitating the study of a wide range of models, including mini-brains (collections of cells able to imitate certain brain structures and functions), zebrafish, and rodents. Increasingly, researchers such as Chris Xu at Cornell University want to push the imaging envelope—and observe, for instance, regions of the mouse cortex and hippocampus associated with complex information processing.
Multiphoton-excited fluorescence microscopy (MPM) is popular for such work because it allows noninvasive, ongoing observation of both the brain's structure and the activity of sensitive brain tissue. Because illumination in the infrared (IR) wavelength range is gentle, it supports sustained imaging over long periods without damage or perturbation of the observed region.
Moreover, various fluorescent proteins are now readily expressed in key animal models through viral infection or genetic expression. Specifically for observation of neuronal activity, scientists leverage genetically encoded calcium indicators (GECIs). GECIs are activity-sensitive indicators that fluoresce in response to an optical excitation when calcium, a proxy for neuronal activity, is present. As with other fluorescent proteins used in multiphoton microscopy, GECIs can be excited with various wavelengths in the IR—for example, 920–950 nm for the GCaMP family and 1000–1100 nm for red-shifted varieties (e.g., jRCaMP1a,b).1
Two- and three-photon imaging
Two-photon excited fluorescence microscopy (2PM) is particularly well suited for video-rate imaging and direct observation of neuronal activity in vivo at depths ranging from tens to hundreds of microns. The excitation source of choice for 2PM is typically a high-repetition-rate femtosecond laser with wide spectral output tunability to cover the IR transparency window in live tissue. Wavelengths between 900 and 1300 nm are particularly important for optimal excitation of key fluorescent proteins, and for achieving decreased scattering in the IR.
Newly introduced laser platforms such as the Spectra-Physics InSight X3 offer high peak-power performance in this range. Thanks to recent power enhancements, this platform is able to outperform legacy Ti:sapphire lasers at wavelengths 900 nm and above, and it has demonstrated its usefulness for two-photon excitation of green, yellow, and red fluorescent proteins (see Fig. 1).
The maximum achievable penetration depth for 2PM is determined by background noise, and is typically limited to 1 mm in live tissue. While this is adequate for imaging most of the mouse cortex, it does not allow imaging of subcortical structures such as the hippocampus, which lie at 1 mm and deeper. To further extend penetration depth, neuroscientists turn to three-photon excited fluorescence microscopy (3PM). Let us briefly examine why.
The 3PM technique relies on a nonlinear process wherein a fluorescent protein simultaneously absorbs three IR photons. Such excitation requires high spatial and temporal photon density that can be realized only in a tight focal volume. This spatially confined optical effect offers background levels several orders of magnitude lower than realized in 2PM. The IR laser excitation wavelength should match the three-photon absorption window of the key fluorescence protein or GECI of interest, and should lie in spectral windows with relatively low water absorption. As demonstrated by Lingyan Shi and colleagues, operating at longer IR wavelengths further reduces optical scattering and extends penetration depth.2 For these reasons, the optimum excitation wavelengths for 3PM are 1.3 μm for green proteins and 1.7 μm for red proteins.
The three-photon absorption process is a low-probability event that requires ultra-high peak power levels to generate fluorescence signal. Compared to 2PM, the instantaneous peak power and photon density must be several orders of magnitude higher, typically in the 1–100 MW range. This boost in peak power is best achieved by increasing the pulse energy to the microjoule level and reducing the temporal pulsewidth to sub 100 fs, while operating with moderate average-power levels (typically tens or hundreds of milliwatts). This ensures compatibility with the imaging of fragile structures—particularly susceptible to heating, phototoxicity, and photodamage associated with excessive average power.3
Highly capable laser tools
One-micron ytterbium ultrafast amplifiers, based on either fiber or diode-pumped solid-state (DPSS) architecture, are well suited to support these needs. They are robust, compact, and fully automated for ease of use. Thanks to their regenerative amplification scheme, they can be operated at various repetition rates and pulse energies to exactly match the application requirements. In the case of 3PM, a repetition rate of 1 MHz and pulse energies of tens of microjoules are typical. An important limitation of ytterbium ultrafast lasers and amplifiers is the lack of spectral tunability, which limits their direct use to applications compatible with 1 μm excitation. To reach the 1.3 and 1.7 μm wavelengths necessary for 3PM, a hybrid collinear/non-collinear optical parametric amplifier (OPA) may be pumped with the second harmonic of the ytterbium amplifier 1 μm emission wavelength (see Fig. 2).Reaching the hippocampus in vivo
Chris Xu and his collaborators at Cornell are pioneers in the development of 3PM and its use for functional imaging deep in the brain. In a recent study, Xu's team demonstrated the optical recording of spontaneous activity from neurons in the hippocampus down to ~1 mm within an intact mouse brain.4 "We are now able to see neurons in action in an intact mouse brain at single-cell resolution and at depths that were not previously possible, which opens new opportunities for neuroscience research," he says.
The work (see frontis, left) was done using a custom-built three-photon microscope and a non-collinear optical parametric amplifier (Spirit-NOPA) pumped by a single-box automated 1 μm ultrafast amplifier (Spirit). "Newly released commercial lasers, such as the Spirit-NOPA system, now offer straightforward tunable output to 1.3 μm and 1.7 μm and enable practical three-photon imaging," Xu comments. "These lasers are easy to operate and to incorporate into an existing multiphoton microscope."
REFERENCES
1. H. Dana et al., eLife, doi:10.7554/eLife.12727 (2016).
2. L. Shi et al., J. Biophoton., 9, 1-2, 38-43 (2016); doi:10.1002/jbio.201500192.
3. J. Klein, "Ultrahigh peak power femtosecond lasers advance bioimaging," BioOptics World, 8, 9, 72-77 (Sept. 2015).
4. D. Ouzounov et al., Nat. Methods, doi:10.1038/nmeth.4183 (2017).
Julien Klein is senior product marketing manager at Spectra-Physics Lasers/MKS Instruments, Santa Clara, CA; e-mail: [email protected]; www.spectra-physics.com.