ULTRAFAST LASERS: Few-cycle pulses create intense attosecond bursts
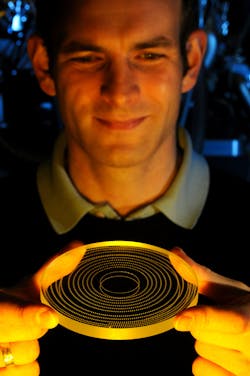
A pan-European team of researchers has succeeded in creating isolated attosecond pulses with unprecedented intensity. The technique opens the way for material-physics investigations using both attosecond-scale pump beams and probe beams. The approach behind the advance makes use of few-cycle, femtosecond-scale pulses to ablate the surface of a mirror, creating a plasma. Two separate but related conversion processes then come into play, with their relative contributions dependent on the input intensity.
One of them, coherent wake emission (CWE), is a recently discovered effect, first put forth by Fabien Quéré of the Department of Research in Condensed Matter, Atoms, and Molecules at CEN Saclay (Gif-sur-Yvette, France) in 2007.1 Coherent wake emission is a kind of “push-pull” effect on the plasma electrons: As the plasma oscillations flow into and out of phase with the input laser’s electric field, they are periodically excited by attosecond electron bunches, resulting in the emission of sub-femtosecond harmonics up to the plasma frequency.
Since that time, such short pulses have been created by exploiting CWE, notably by a collaboration led by researchers at the Max Planck Institute for Quantum Optics (MPQ; Garching, Germany), who recently used extreme-UV (XUV) autocorrelation to get a full temporal characterization of their sub-femtosecond pulses.2
Relativistic oscillating mirror
However, at a threshold intensity, another effect becomes dominant: The relativistic oscillating mirror, or ROM. The ROM effect occurs when the intense input field drives the surface of the plasma, causing electrons to oscillate at relativistic speeds. The periodic phase modulation in the reflected beam gives rise to harmonics of the laser frequency, and the conversion process can lead to higher output photon energies than CWE.
Now the MPQ researchers, in collaboration with researchers at the Foundation for Research and Technology Hellas (Crete, Greece) and Queens University Belfast (Belfast, Ireland) are pushing ROM to its limit, demonstrating the first relativistic harmonic generation triggered by few-cycle pulses. The team used a laser source based on noncollinear optical parametric chirped-pulse amplification to create input pulses of 8 fs duration—just three cycles of the field—and 16 TW of peak power, incident on a fused-silica blank as the solid-target source of the plasma.
The researchers found that the output neatly matched their particle-in-cell simulations of the ROM process: For 17% of the input pulses, a single XUV attosecond-scale pulse was created, with a wavelength as small as 17 nm. About a third of the time, a pair of pulses was produced, and half the time, a triplet.3 The team estimates that future improvements to their laser, bringing it down to a 5 fs pulsewidth, will result in a greater than 50% chance of single pulses.
Moving target
Patrick Heissler, lead author of the study, believes the method holds more promise for future attosecond pulse sources than more established methods. “The approach to isolated attosecond pulse production we demonstrate promises unprecedented attosecond pulse intensities,” he says. “Compared to state-of-the-art attosecond pulse sources based on gas harmonics, higher conversion efficiencies into the XUV are predicted. Due to the direct use of the plasma medium, no limitations on the laser pulse intensities are implied and hence a high-intensity driving laser can be efficiently exploited.”
However, the creation of the plasma that drives the whole technique is inherently a destructive process (see figure). “A very clean interaction surface is necessary but the target surface is destroyed with every laser shot. Hence, a fresh piece of target needs to be provided for every new laser pulse,” says Heissler. “In current systems, the used glass target is rotated and/or shifted to provide a clean surface, allowing repetition rates on the order of kilohertz. For future experiments, new target systems like tape drives or liquid jets need to be developed.”
Once target issues are addressed, though, the only limiting factor in the XUV photon energy and attosecond pulsewidth at the output is the intensity and pulsewidth of the input laser.
REFERENCES
1. C. Thaury et al., Nat. Phys., doi:10.1038/nphys595 (2007).
2. R Hörlein et al., New J. Phys., 12, 043020 (2010).
3. P. Heissler et al., Phys. Rev. Lett., 108, 235003 (2012).
About the Author
D. Jason Palmer
Freelance writer
D. Jason Palmer is a freelance writer based in Florence, Italy.