PHOTONIC FRONTIERS: VCSELS: Vertical cavity lasers reach higher speeds and powers
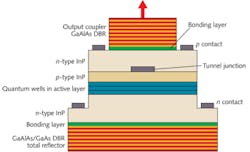
Invented in 1977 by Kenichi Iga, vertical-cavity surface-emitting lasers (VCSELs) were a radical departure from the edge-emitting design used in the first semiconductor diode lasers. Edge emitters oscillate in a cavity stretching hundreds of micrometers or more along the plane of a diode junction, generating a high-divergence beam that emerges from a small area on the cleaved edge of the chip. Vertical cavity lasers oscillate perpendicular to the junction plane, so the resonator contains only a thin layer of gain medium and requires high-reflectivity mirrors to sustain oscillation, but they have low threshold currents and generate a better-quality, lower-divergence beam.
Those qualities long defined VCSELs as efficient but low-power devices that were less expensive to package and produce than edge emitters. That made VCSELs natural choices for low-power applications such as short-distance fiber-optic data communications and motion sensing in computer mice. Now new technology is making VCSELs far more versatile by increasing modulation speed, output power, and the range of wavelengths available.
Wavelengths and materials
Distributed Bragg reflectors (DBRs) located above and below the junction layer form resonant cavities in VCSELs. This works very well in gallium aluminum arsenide (GaAlAs), because the refractive indexes of GaAlAs and GaAs differ enough to make good DBRs, and VCSELs have long been important sources for short fiber links in the 850 nm fiber window. Refractive indices do not differ enough, however, to make good DBRs in the indium phosphide (InP) compounds that emit in the 1300 and 1550 nm windows. That and problems in fabricating current-confinement structures stalled development of long-wavelength VCSELs, wanted for transmitting high-speed signals through 10 to 20 km of standard singlemode fiber for access networks.
Now new techniques are successfully producing long-wavelength VCSELs. Strained InP/InAlGaAs quantum wells allow fabrication of DBRs containing alternating layers of InAlGaAs and InAlAs on InP substrates. That combination has yielded milliwatt-class output, limited by the poor heat dissipation of InAlGaAs. Replacing the total reflector with a dielectric DBR improves head conduction, allowing higher power. Another alternative is wafer-fusing AlGaAs/GaAs DBRs to the top and bottom of an active cavity of InAlGaAs/InP with an internal regrown tunnel junction that improves current control—a technique Beam Express (Lausanne, Switzerland) uses to make 1310 nm VCSELs (see Fig. 1).1
Another new approach is the use of high-contrast gratings, developed by Connie Chang-Hasnain’s group at the University of California–Berkeley (Berkeley, CA). The single-layer structure is essentially a grid of thin subwavelength stripes of high-index material such as silicon surrounded by a low-index medium such as air or silica. Fabricated by photolithography, the high-contrast grating is only about one-fortieth the thickness of the DBR structures normally used in VCSELs but reflects 99.5% of light orthogonal to the grating plane. Suspended above the active layer, it is less than one-thousandth the weight of standard microelectromechanical (MEM) mirrors and can tune the VCSEL wavelength rapidly (see Fig. 2).2After demonstrating the concept at 850 nm, the Berkeley group moved to longer wavelengths. In 2010, it demonstrated a 1550 nm VCSEL with a high-contrast grating and an enhanced current-control structure that emitted more than 1 mW continuous wave at room temperature, and operated at temperatures up to 60°C.3 Varying the size of the air gap in an array produced lasers emitting at fixed wavelengths from 1540 to 1591 nm that are promising for wavelength-division multiplexing.4
High-speed VCSELs
Today’s storage area networks and data centers use 850 nm VCSELs to transmit data at 10 Gbit/s through up to 100 m of multimode graded-index fiber. Small and efficient, VCSELs can be packed closely together in transmitters, but system developers want even faster speeds and lower power consumption—measured in femtojoules per bit—to serve tomorrow’s massive data centers. The first step up will be to fiber line rates of 25 Gbit/s, so four wavelengths can carry 100 Gbit/s. With further refinements, writes Anders Larsson of Chalmers University of Technology (Göteborg, Sweden) in a review paper, it is likely “present high-speed VCSEL technologies (at both short and long wavelengths) will be capable of 40 Gbit/s modulation over a wide range of temperatures and with the margins needed in real systems. However, it is also likely that 40 Gbit/s is the upper limit for direct current modulation. Reaching a higher single channel speed (such as 100 Gbit/s) will require new innovative modulation schemes and/or new modulation formats.”5
At of the start of this year, GaAs-based VCSELs had reached data rates of 40 Gbit/s at 850 and 1060 nm, and 35 Gbit/s at 980 nm. The longer wavelengths offer two key advantages over 850 nm. High-temperature performance tends to be better at longer wavelengths, with 25 Gbit/s recorded at temperatures to 85°C at 850 nm, but to 100°C at 1060 nm. A 980 nm laser reached 120°C but only at 20 Gbit/s. Perhaps more important, the longer-wavelength VCSELs consume less energy per bit at high speeds—as little as 240 fJ/bit at 1060 nm and 285 fJ/bit at 980 nm, compared with a minimum of 330 fJ/bit at 850 nm. With heat dissipation a crucial issue for high-performance computing, femtojoules matter.
Even better results have been reported in 2011. At Photonics West in January, a team from Furukawa Electric (Ichihara, Japan) reported power dissipation of only 140 fJ/bit when transmitting 10 Gbit/s at 1060 nm.6 At the Optical Fiber Communication Conference in March, W. Hofmann of the Technical University of Berlin (Berlin, Germany) and colleagues reported error-free transmission of 44 Gbit/s with 980 nm VCSELs operated at 25°C using less than 300 fJ/bit. At 85°C, where the highest reported speed had been 25 Gbit/s, they recorded an impressive 38 Gbit/s. “This means that serial bit rates of 40 Gbit/s in un-cooled, un-monitored conditions at high power efficiencies below 300 fJ/bit are feasible,” they wrote.7
So far, 1300 and 1550 nm VCSELs lag somewhat in data rate. Larsson’s review listed one report of 25 Gbit/s at 1550 nm, but the next highest was 12 Gbit/s.
High-power VCSELs
What counts as high power depends on the type of VCSEL. Producing the singlemode output needed for many applications requires limiting the emitting aperture to a few micrometers, and that small size limits singlemode continuous-wave emission to milliwatt powers on all bands for flat-reflector monolithic VCSELs. Further refinements in efficiency and thermal management should allow powers to exceed 10 mW at both short and long wavelengths, Larsson writes, but dramatic increases are unlikely.
Scaling VCSELs to much larger emitting areas or fabricating two-dimensional arrays can generate much higher powers in multimode beams. These devices spread the power over a large enough area to avoid optical damage to the surface, and can operate in pulsed, quasi-continuous-wave, and continuous-wave modes. (Optically pumped vertical external cavity lasers, or VECSELs, also can generate higher powers, but they are not diode lasers.)
Power levels from large lasers have been climbing. In April, a team from the Changchun Institute of Optics, Fine Mechanics, and Physics (Changchun, Jilin, China) reported record peak power from bottom-emitting 980 nm lasers with 500 µm apertures.8 Grown on a GaAs substrate, the lasers include an n-type DBR, an active layer containing three InGaAs quantum wells, and a top mesa containing a p-type DBR with 99.9% reflectivity (see Fig. 3). The output coupler is 99.3% reflective. Threshold current was 0.8 A, with maximum continuous-wave output of 1.62 W at room temperature and drive current of 4.5 A. Quasi-CW operation reached 2.89 W at 6 A current, limited by the power supply. When driven with 60 ns pulses of 110 A, peak power reached 92 W, a record for a single device.Larger arrays of smaller devices can generate more power because efficiency of single VCSELs decreases with their aperture, and heat dissipation becomes difficult. Power from multiple arrays can reach kilowatt levels. At Photonics West in January, Philips Research Laboratories (Aachen, Germany) reported testing a prototype 2 kW VCSEL source for more than a year.9 They found that relatively simple micro-optics and field lenses, together with tailoring shapes of individual VCSELs, could make illumination uniform within about 1%.
Outlook
Vertical cavity lasers have become remarkably versatile. Developers have moved beyond GaAs, not just to InP compounds, but also to nitrides at blue wavelengths and antimonides near 2 µm. These devices are being developed for applications ranging from tiny, high-efficiency milliwatt lasers for sensing to kilowatt-class lasers for heating and drying. They are being fine-tuned for critically demanding applications such as data links within server farms, where shaving femtojoules from switching energies is important. And the field is moving fast enough that by the time you read this article, something in it is likely to be outdated.
REFERENCES
1. E. Kapon and A. Sirbu, “Long-Wavelength VCSELs: Power efficient answer,” Nature Photon., 3, 27 (January 2009).
2. C. Chang-Hasnain et al., “High-Contrast Grating VCSELs,” IEEE J. Sel. Top. in Quant. Electron., 15, 3, 869 (May/June 2009).
3. C. Chase et al., “1550 nm high contrast grating VCSEL,” Opt. Exp., 18, 15, 15461 (July 19, 2010).
4. Y. Rao, C. Chase, and C.J. Chang-Hasnain, “Multiwavelength HCG-VCSEL array,” IEEE Intl. Semiconductor Laser Conf. 2010, Technical digest, 11–12; doi: 10.1109/ISLC.2010.5642778.
5. A. Larsson, “Advances in VCSELs for Communication and Sensing,” to be published in IEEE J. Sel. Top. in Quant. Electron. advance online publication; doi: 10.1109/JSTQE.2011.2119469.
6. K. Takaki et al., “1060 nm VCSEL for intra-chip optical interconnection,” Proc. SPIE, 7952, 795204; doi: 10.1117/12.873662.
7. W. Hofmann et al., “44 Gb/s VCSEL for optical interconnection,” OFC 2011, PDPC5.
8. L. Zhang et al., “High-power bottom-emitting Vertical-cavity surface-emitting lasers under continuous-wave, quasi-continuous-wave, and pulsed operation,” Appl. Phys. Exp., 4, 052102 (Apr. 19, 2011).
9. H. Moench et al., “High power VCSEL systems for tailored intensity distributions,” Proc. SPIE, 7952, 795207; doi: 10.1117/12.873813.
About the Author
Jeff Hecht
Contributing Editor
Jeff Hecht is a regular contributing editor to Laser Focus World and has been covering the laser industry for 35 years. A prolific book author, Jeff's published works include “Understanding Fiber Optics,” “Understanding Lasers,” “The Laser Guidebook,” and “Beam Weapons: The Next Arms Race.” He also has written books on the histories of lasers and fiber optics, including “City of Light: The Story of Fiber Optics,” and “Beam: The Race to Make the Laser.” Find out more at jeffhecht.com.