PHOTONICS APPLIED: BIOPHOTONICS: Molecules can't hide from the newest in-vivo imaging techniques
The in-vivo optical molecular imaging market will reach $400 million in 2014 and nearly a billion dollars by the end of 2020, according to Strategies Unlimited (Mountain View, CA). Already, numerous companies have moved optical molecular imaging technology out of the laboratory and into preclinical settings, with promises of revolutionary disease diagnosis, treatment, and cure.
Defining OMI; making mice glow
Optical molecular imaging (OMI) can be loosely defined as the science of visualizing chemical processes on the molecular level. The toolset for obtaining the visual image is typically a low-cost optical microscopy or simple imaging setup. Chemical probes or agents induce fluorescence or some other form of "visible" signature in the molecule for real-time observation, and images can be combined or overlaid with data from additional imaging modalities. Still other OMI methods use spectroscopic signatures alone to distinguish certain molecules from others—a technique called "label-free" imaging.
Fluorescent proteins such as GFP (green fluorescent protein; originally harvested from jellyfish and cloned) and bioluminescence (proteins harvested from fireflies, for example) are extremely useful as research tools to better understand protein and enzyme interactions and degradation mechanisms in living cells and tissue. Dyes can be attached to particular antibodies, peptides, or small molecules specifically targeted to particular cells. Activatable agents can be designed to degrade in the presence of enzymes involved in metastatic processes, resulting in a fluorescent signal. Alternatively, tumor cells can be engineered to express genes for fluorescent proteins, enabling internal cellular processes to be monitored.
Both fluorophores and genetically engineered proteins have their pros and cons. "If you dye your hair, you have to repeat the process because newly growing hair will not contain the dye. But genes are forever: You can link GFP to other proteins or genes that control cell division, metabolism, or express hair color, and the gene can be there for the life of the animal," says AntiCancer (San Diego, CA) CEO Robert Hoffman. "We've even developed a mouse with GFP green hair; genetic human hair-color treatments may be in our future."
An early pioneer of "glowing mice," Hoffman's company was the first to use GFP for in-vivo small-animal imaging (see Fig. 1).1 AntiCancer now markets MetaMouse—a line of naturally immune-deficient nude mice that contain bits of GFP-expressing or other colored-protein-expressing human tumor tissue for in-vivo functional imaging. "Better still, these glowing mice can be imaged with a $27 excitation lamp and a $150 optical filter; the beauty of OMI is its accessibility to a wide range of researchers," he says.
Although genetically engineered agents such as GFP do not yet have FDA approval, the inert dye indocyanine green (ICG) is currently FDA-approved for human clinical trials. "GFP is extremely important for studying how a disease progresses once an animal is transformed to glow; however, you don't want to genetically engineer patients. Instead, you want to find disease that is already there," says Spectros (Portola Valley, CA) CEO David Benaron, a founder of several OMI-related startups. "In humans, the opportunities are just now moving from the laboratory to the clinic. Fluorophores designed to concentrate and report from inside the body in the presence of a disease can actually tell clinicians whether or not a subject has that disease and where it is concentrated," Benaron adds.
Multiple modalities
"Depth penetration of light is still the primary obstacle to applying OMI to humans; we simply cannot penetrate more than 5 or 6 cm or so into human tissue for in-vivo studies," says Dr. Seth Gammon, worldwide in-vivo product manager for Carestream Health (Woodbridge, CT). "But progress is being made; in short, OMI is the fastest path to disease diagnosis and cure that is available today, using way fewer mice than other more invasive or radiation-intensive technologies."
Gammon explains that diagnostic and surgical applicability of OMI to humans is practical using catheters or endoscopic probes. For example, a fluorescent probe designed to report tissue oxygenation can distinguish between live and dead tissue to guide a surgeon. And AntiCancer demonstrated that an engineered adenovirus containing GFP can label metastatic human tumors growing in mice for fluorescence-guided surgery.2
Carestream Health, which has its roots as the spinoff of Kodak's molecular imaging group, introduced its Kodak In-Vivo Multispectral Imaging System FX to output visible reflectance, multiwavelength fluorescence, radioisotopic, and x-ray images in a single 20 × 20 cm field of view with 4 Mpixel resolution, providing molecular and structural information simultaneously (see Fig. 2). With spectral deconvolution, the system can filter 29 different excitation wavelengths between 390–770 nm from its 175 W xenon source.All OMI system manufacturers are careful to minimize the effects of autofluorescence. "We have many different chromophores in our skin and tissue that fluoresce naturally. This background fluorescence is not a problem in vitro when you're working with a layer of cells, but its cumulative effects in vivo are significant," says Gammon. "Our imaging systems minimize autofluorescence by analyzing the shape of the spectral excitation signatures and by subtracting those signatures from an agent-free control animal."
Targeting disease in 3D and over time
VisEn Medical (Bedford, MA), which offers more than 35 unique in-vivo fluorescent agents, takes spatial imaging a step further. "There is a natural scattering of photons by biological tissue that causes a conventional 2D signal at the surface of an object to lose correlation with the true fluorescent signal emanating from the true target," says Rob Sandler, senior vice president of marketing at VisEn. "The FMT platform uses lasers to transilluminate the object from multiple positions on one side, with a camera on the opposite side to collect both laser excitation and agent fluorescence information. It then models light propagation through the tissue, acquiring sufficient depth information to calculate the exact location and concentration of fluorescence within the 3D space of the object. This capability is important in most research areas where deep-seated anatomies, like tumor metastasis in orthotopic sites, can clearly be monitored and quantified," adds Sandler (see Fig. 3). "Respiratory diseases such as COPD are also manifested in deep anatomical targets and the FMT can easily visualize and quantify respiratory biomarkers in vivo."Caliper Life Sciences' (Hopkinton, MA) director of imaging marketing Stephen Oldfield is seeing the benefits of OMI for disease diagnosis and drug discovery first-hand. "Caliper now has a dominant share of the preclinical OMI market and continues to develop the technology in areas like 3D imaging and quantification," says Oldfield. "As of mid-March 2010, the bibliography of peer-reviewed publications using Caliper's IVIS systems now runs to 1433 publications." IVIS systems use fluorescent protein or bioluminescent reporters to reveal biological processes like gene expression, tumor growth, infection spread, or circulatory function, and are even sensitive enough to visualize the light emitted from radioactive tracers—particularly those used in clinical molecular imaging.3
The IVIS Spectrum uses laser light to generate a 3D surface map of a mouse and a filtered white light source for fluorescence excitation to give a broad range of wavelength options. Using transillumination, a fluorescent protein such as tdTomato can be easily imaged in a deep tissue such as the prostate. With spectral unmixing, an antibody like trastuzumab labeled with a long-wavelength dye such as CF750 can be imaged and quantified at the cell surface. Gene expression is easily monitored using bioluminescence, so in a transgenic animal the development of new blood vessels can be monitored in conjunction with tumor development. And the IVIS Lumina XR can explore rheumatoid arthritis using a fluorescent bone probe, a bioluminescent inflammation probe, and x-ray images for colocalization.
Both fluorescent and bioluminescent signals can be visualized in 3D using IVIS tomographic techniques, and 3D reconstructions can be coregistered with other imaging modalities. All IVIS systems use a -90°C cooled CCD and a choice of filter options for spectral unmixing. The cooled CCD minimizes dark current and provides the highest signal-to-noise ratio for sensitive imaging of bioluminescent signals: IVIS can detect a single cell under the skin of a mouse.
With 20 years of experience in fluorescence imaging, CRi (Woburn, MA) is bringing multispectral "kinetic imaging" to the OMI field. Its Maestro multispectral systems easily remove autofluorescence in skin tissue from the fluorescence signal of a labeled fluorophore and incorporate a liquid-crystal tunable filter—the equivalent of 200 bandpass emission filters—to distinguish between different fluorophore signals within an animal. And DyCE (Dynamic Contrast Enhancement) technology from CRi combines rapid imaging (up to 15 frames/s monochrome or 10 s/frame multispectrally) with an advanced data processing methodology that allows the determination of the rates of change of the intensity of the fluorophore in each pixel of the image and the rate of uptake and wash-out in the animal. By utilizing this data, a much higher-contrast image of the accumulating fluorophore can be obtained in a much shorter period of time—minutes and hours versus days (see Fig. 4).Coming full circle
LI-COR Biosciences is also using OMI to target disease. Its Pearl Impulse Imager has been used with a variety of different contrast agents based on their near-IR dye IRDye 800CW for identifying tumor tissue, metastasis, vasculature, lymphatic structure, and metabolic activity.4,5 "LI-COR started working on near-IR technology back in 1971, probably earlier than many other companies," says Michael Olive, vice president of translational research at LI-COR Biosciences (Lincoln, NE). But he explains that OMI is just now gaining traction for several reasons: 1) the availability of near-IR fluorophores and other contrast agents; 2) improvements in available wavelength-selective and low-cost broadband and laser light sources and detectors; and 3) size reductions in available components that make in-office, portable imaging instrumentation possible. "I have trouble programming a VCR," says Olive. "The fact that I can take our Pearl Impulse Imager out of the box and have it working in no time is incredible." He concludes, "All of these enabling factors have finally come together into a ‘perfect storm'; 2010 is definitely the year for optical molecular imaging."
REFERENCES
- T. Chishima et al., Cancer Research 57, pp. 2042–2047 (May 15, 1997).
- H. Kishimoto et al., Proc. Nat'l. Acad. Sciences (PNAS) 106, 34, pp.14514–14517 (Aug. 25, 2009).
- H. Liu et al., "Molecular Optical Imaging with Radioactive Probes," Online access journal from the Public Library of Science, PLoS ONE 5, 3, e9470 (Mar. 1, 2010).
- J. Kovar et al., American J. Pathol. 169, pp. 1415–1426 (2006).
- J. Kovar et al., Analytical Biochem. 384, pp. 254–262 (2009).
Label-free imaging
A new broadband coherent anti-Stokes Raman scattering (CARS) microscopy/spectroscopy method recently developed at the University of Wisconsin–Milwaukee depends not on a chemical agent, but on unique differences in the vibrational spectra of several molecules to aid in disease diagnosis and detection. This label-free (does not require fluorescent labels or probes) method uses a neodymium:yttrium vanadate (Nd:YVO4) laser for both pump and probe radiation and a broadband continuum generated in a special germanium-doped fiber as the Stokes pulse to spectrally distinguish between omega-3 (unsaturated) and omega-6 (saturated) fatty acids in the blood. The method can also easily distinguish calcium oxalate (COX) microcalcifications typically present in healthy breast tissue from calcium hydroxyapatite (HAP) microcalcifications often present in breast tissue that later show malignancy—something that brightfield or other visual imaging techniques cannot do.
Another label-free alternative OMI technique from Harvard University uses a method called stimulated emission microscopy. A particular wavelength of light excites hemoglobin in the blood, enabling 3D mapping of blood-oxygenation levels and revealing details as small as single red blood cells in individual capillaries.
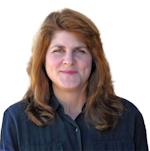
Gail Overton | Senior Editor (2004-2020)
Gail has more than 30 years of engineering, marketing, product management, and editorial experience in the photonics and optical communications industry. Before joining the staff at Laser Focus World in 2004, she held many product management and product marketing roles in the fiber-optics industry, most notably at Hughes (El Segundo, CA), GTE Labs (Waltham, MA), Corning (Corning, NY), Photon Kinetics (Beaverton, OR), and Newport Corporation (Irvine, CA). During her marketing career, Gail published articles in WDM Solutions and Sensors magazine and traveled internationally to conduct product and sales training. Gail received her BS degree in physics, with an emphasis in optics, from San Diego State University in San Diego, CA in May 1986.