PHOTONIC FRONTIERS: SHORTWAVE LASER DIODES: The quest for practical green laser diodes
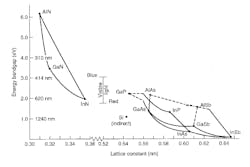
The quest for shorter laser-diode wavelengths began with efforts to push gallium-arsenide emission from the infrared (IR) to visible red wavelengths, eventually yielding the now common red laser diodes, with active layers of gallium-indium phosphide (GaInP) or aluminum gallium indium phosphide (AlGaInP) on gallium arsenide (GaAs) substrates. The next step was a big one, into the blue, when Shuji Nakamura succeeded in making 405 nm laser diodes from GaInN in 1995.
Blue nitride laser diodes have proved to be a brilliant success, but they left a gaping green hole in the middle of the laser-diode spectrum. That's become a problem as new applications have emerged for true green laser diodes, particularly in full-color laser displays. Frequency doubling of neodymium (Nd) lasers to 532 nm can fill the color gap temporarily, but display developers want green laser diodes because they are more compact, can be directly modulated, and have a broader bandwidth that suppresses laser speckle—a potential distraction in a visual display. Laboratory demonstrations now have reached the green, but the quest for practical green laser diodes remains a technological horse race.
From blue to green
Green laser diodes require larger direct bandgaps than are available from arsenides and phosphides, but GaInN can be made to emit in the 520-530 nm band needed for good color balance with red and blue laser diodes (see Fig. 1). Unfortunately, reducing the active-layer bandgap enough to reach the 520-530-nm band requires increasing the indium concentration (compared to gallium) to about 30%, giving Ga0.7In0.3N.
That's a problem because increasing indium concentration in the active layer above 15% makes the semiconductor crystal more prone to defects that impair laser operation. That difficulty stalled the push from blue to the longer (488 nm) wavelengths through 2008. In early 2009, developers finally broke through that barrier to demonstrate GaInN laser diodes emitting at 500 nm. Two different approaches to growing laser structures on GaN substrates succeeded at about the same time, and both are still in the running.
Gallium nitride normally forms a hexagonal wurtzite crystal that usually serves as a substrate for GaInN laser diodes. Most blue and violet laser diodes are grown on the c-plane containing the hexagonal structures (see Fig. 2). That choice is convenient, but the polarity inherent in the crystal produces large internal electric fields perpendicular to the c-plane, and those fields separate the wave functions of electrons and holes in quantum wells grown in the nitride compound. That separation—called the quantum-confined Stark effect—reduces the recombination rate, and thus decreases light-emission efficiency in laser diodes and LEDs.1 However, that separation also reduces the energy of photons emitted during recombination, increasing the wavelength from the blue without adding more potentially troublesome indium. Osram Opto Semiconductors (Regensberg, Germany) used this approach to reach 500 nm.2
Other GaN crystal planes, also shown in Fig. 2, have lower or no polarity, so an alternative is to grow laser structures on those planes. Planes on the sides of the hexagonal structure, called m-planes, are nonpolar but can be difficult to fabricate. Planes cut at a 45° angle to the c-plane and the crystal axis are called semipolar, because their polarity is lower than the c-plane, and they are easier to fabricate. Growing lasers on a semipolar or nonpolar plane keeps the wave functions of electrons and holes closely aligned, increasing the recombination rate and thus increasing the light-emission efficiency. On the other hand, it requires more indium to reach the desired wavelengths. The Rohm Co. (Tokyo, Japan) used this approach to reach 500 nm shortly before Osram.3These two approaches are the leading contenders in a horse race that has pushed from the blue to longer wavelengths near 525 nm.
Low-polarity substrates
Both Rohm and Nakamura's group at the University of California at Santa Barbara (Santa Barbara, CA) tested their ideas for low-polarity substrates by making blue laser diodes. After showing that low-polarity substrates improved efficiency and output power of blue lasers, Nakamura, James Speck, and Steven DenBaars formed Kaai Inc. (Goleta, CA) in early 2008 to develop that technology for laser diodes. This year they merged Kaai into a related venture, Soraa Inc. (Goleta, CA).
So far, they have made 405 nm violet laser diodes emitting up to 0.5 W singlemode with 25% wall-plug efficiency, and blue diodes emitting up to 0.75 W singlemode, with efficiency reaching 23–24%. They say both efficiencies are several percent higher than for c-plane substrates, but their real goal is green laser diodes. "It only gets tougher as you get to longer wavelengths and add more indium," says Paul Rudy of Soraa. Low-polarity substrates allow electrons and holes to be closer to each other, giving designers more flexibility in choosing materials for their lasers, Rudy says, but he would not disclose what materials Soraa is using, or whether the substrates are nonpolar or semipolar.
After reporting a 523 nm laser at Photonics West in January 2010, Soraa (as Kaai then) sought to increase power and efficiency rather than pushing to 532 or 535 nm. "We are now up to 60 mW, and focused mainly on the 520–25 nm range," Rudy says. That's roughly a factor-of-10 improvement in continuous-wave power from early in the year. Wall-plug efficiency is only 2%, but Soraa is pushing for improvements. "You need 8% wall-plug efficiency for commercial success, and we think more than 10% is possible in the next year," says Rudy. As promised at Photonics West, Soraa has begun shipping sample green lasers.
Reliability is a key issue. Soraa only recently began preliminary life tests of green laser diodes, but tests of blue lasers on low-polarity substrates have passed 10,000 h. So far the green results look similar, but Rudy wouldn't discuss details.
C-plane polar substrate lasers
Advocates of polar substrates see a different balance—reducing electron-hole overlap also reduces photon energy to give longer wavelengths with lower indium concentrations. Adrian Avramescu and colleagues at Osram calculate that growing 3 nm quantum wells on polar GaN substrates would reduce the amount of indium needed for 531 nm lasers to 30% from 34% on nonpolar GaN (see Fig. 3).4 Quantum-well thickness also matters. Increasing the thickness of quantum wells from 2.5 to 3 nm reduces indium concentration required to produce a given wavelength by about 1.5%.Increasing the quantum well thickness carries a tradeoff, however, because it also decreases the overlap of electrons and holes inside polar quantum wells, which decreases recombination rate and model gain. "Polar designs are a tradeoff between low indium concentration of 4 nm wide quantum wells and high overlap of electrons and holes in 2 nm narrow designs," write Uwe Strauss and colleagues at Osram in a paper online at Physica Status Solidi B.5 Nonetheless, they conclude, "the compromise on [the] c-plane still shows the best device performance."
In experiments with broad-area c-plane lasers operated at 25°C, Osram demonstrated pulsed laser operation at 531.7 nm with a 6% duty cycle. From those experiments, they concluded that the limiting factor for green lasers was the crystal quality in high-indium GaInN quantum wells, not the high polarity of the c-plane substrates. A continuous-wave ridge laser emitting at 524 nm reached peak wall-plug efficiency of 2.3% at 50 mW power.
Outlook
It's the kind of horse race that's both exciting to watch and good for the field. Other groups including Sumitomo Electric (Itami, Hyogo, Japan) and Nichia (Tokushima, Japan) also have reported green diodes (see table). Sony also has said it's working on green diodes but has provided few details.Promising new ideas are in the works. Elias Towe of Carnegie Mellon University (Pittsburgh, PA) argues that the best substrate would be the ternary compound Ga0.85In0.15N. In addition to working for green laser diodes, it also could serve as a substrate for red and blue lasers.6 Thus the red, green, and blue lasers needed for a color projector could be fabricated on a single monolithic substrate, potentially making them simpler and less expensive. That's an intriguing possibility for the future.
The near-term goal is to improve performance of green laser diodes and provide the power and reliability needed for displays and other applications that would benefit from the eye's high sensitivity to green light. To achieve those goals, "the most important activity is to improve [internal quantum efficiency] in the green" beyond the present 30–40%, write Hiroaki Ohta, DenBaars, and Nakamura of UC Santa Barbara in a new paper on the future of green laser diodes.7
REFERENCES
1. J.S. Speck and S.F. Chichibu, "Nonpolar and Semipolar Group III Nitride-Based Materials," MRS Bulletin, 34, 304-313 (May 2009).
2. D. Queren et al., Appl. Phys. Lett., 94, 081119 (2009).
3. K. Okamoto, J. Kashiwagi, T. Tanaka, and M. Kubota, "Nonpolar m-plane InGaN multiple quantum well laser diodes with a lasing wavelength of 499.8 nm," Appl. Phys. Lett., 94, 071105-1-071105-3 (2009).
4. A. Avramescu et al, "True green laser diodes at 524 with 50 mW continuous wave output power on c-plane GaN," Appl. Phys. Exp., 3 061003 (2010).
5. U. Strauss et al., "Pros and cons of green InGaN laser on c-plane GaN," Physica Status Solidi B, doi: 10.1002/pssb/201046299 (2010).
6. T.K. Sharma and E. Towe, "On ternary nitride substrates for visible semiconductor light emitters," Appl. Phys. Lett., 96, 191105 (2010).
7. H. Ohta, S.P. DenBaars, and S. Nakamura, "Future of group III nitride semiconductor green laser diodes," J. OSA B, 27 B45 (November 2010).
About the Author
Jeff Hecht
Contributing Editor
Jeff Hecht is a regular contributing editor to Laser Focus World and has been covering the laser industry for 35 years. A prolific book author, Jeff's published works include “Understanding Fiber Optics,” “Understanding Lasers,” “The Laser Guidebook,” and “Beam Weapons: The Next Arms Race.” He also has written books on the histories of lasers and fiber optics, including “City of Light: The Story of Fiber Optics,” and “Beam: The Race to Make the Laser.” Find out more at jeffhecht.com.