LASER PHYSICS: ‘Light shining through walls’ experiment gets a boost
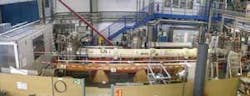
In a small experiment at the DESY synchrotron source (Hamburg, Germany), a detector is effectively staring at a wall, waiting for light to come through. The experiment is called ALPS, for Axion-Like Particle Search, and if a photon should make its way through, we could learn a lot about the nature of dark matter—which is postulated to make up the bulk of the universe’s mass, but whose apparent existence is a continuing mystery to theorists.
The current Standard Model of physics describes nearly all particle interactions that can be imagined. A number of theoretical constructs have been invoked to solve the dark-matter problem, including weakly interacting massive particles, or WIMPs. As their name implies, they don’t interact much with matter, making them difficult to detect by conventional means.
On the other end of the scale are incredibly light particles, or of “sub-eV” mass in particle-physics parlance. WIMPs’ lighter cousins are accordingly called WISPs, and one potential dark-matter recipe could have them playing a major role. The ALPS team believes they might catch sight of a WISP by staring at a wall, on the other side of which is a high-power laser.
Photons to WISPs and back?
The idea is simple: theoretically, but very rarely, a photon could turn into a WISP, which would not interact with matter in any appreciable way and therefore could not be detected conventionally.
Equally, a WISP could convert back into a photon. Rare though it is, the process could be happening all the time but detectors simply wouldn’t see the interim process. The ALPS experiment is a type of tunneling phenomenon, which counts on the possibility that a photon could not pass through a wall but a WISP could.
Should it turn back into a photon on the other side of the wall, the photon could be detected, thus proving that in the meantime, a WISP had been created. It sounds unlikely, but one kind of WISP has already become part of the standard model: the neutrino.
“Neutrinos were postulated and confirmed as subtle effects in laboratory experiments,” the authors note. “Nowadays, they are essential ingredients in our understanding of cosmology and astrophysics.”
While the highest energy regimes are probed by using ever-larger particle accelerators—notably the Large Hadron Collider in Switzerland—to hunt for as-yet unseen particles, the ALPS team argues that, equally, a “hidden sector” could prove instructive by looking for the subtle effects in decidedly smaller experiments. Laboratory experiments at the low-energy frontier are therefore complementary to collider efforts in the search for an accurate description of nature, they say.
The team’s so-called “light shining through walls” experiment makes use of the output from a nonplanar ring oscillator operating continuous wave at 1064 nm, amplified by four diode-pumped Nd:YAG amplifiers. The output is frequency doubled, resulting in an 800 mW output at 532 nm.
Because WISPs should occur in the presence of a high magnetic field, the beam is then passed through a vacuum tube embedded in one of the magnets from DESY’s HERA experiment, providing a magnetic field of 5 T.
Optical resonator
To increase the average power inside the magnetic field and increase their chances of observing a WISP, the researchers have recently implemented a simple optical resonator within the vacuum tube, allowing the beam to remain in the cavity. The team measures an average power in the cavity of about 34 W.
On the other side of the cavity mirror, in the center of the 10-m-long magnetic chamber, is an absorber. On the other side of that absorber is a commercial, cooled CCD.
The team has published its first calibrations and some suggestions for improvements to the experimental sensitivity by way of improvements to the resonant cavity.1 But beyond that, it truly is a matter of staring at the wall and waiting for a chance to change our understanding of particle physics.
REFERENCE
- arXiv preprint server, arXiv:0905.4159v1.
D. Jason Palmer | Freelance writer
D. Jason Palmer is a freelance writer based in Florence, Italy.