PHOTONIC FRONTIERS: PHOTONIC CRYSTALS: Photonic crystals foster a burst of innovation
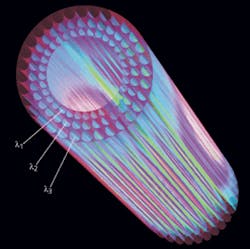
Photonic crystals are coming of age. The 1987 Physical Review Letters paper in which Eli Yablonovitch introduced the concept has been cited more than 5000 times.1 The field’s first textbook is now out in a second, extensively revised, edition.2 A quick search of the agenda of last year’s CLEO/QELS Conference in San Jose turned up the term in the titles of 63 papers and five session titles; nine other paper titles included the phrase “photonic-bandgap fiber.” Significantly, most papers weren’t in sessions on photonic-crystal concepts. Instead, they appeared in sessions ranging from “novel techniques in beam shaping and sensing” to gallium nitride lasers.
The key concept behind photonic crystals is light confinement, but the concept is more than redefining a multilayer dielectric filter as a one-dimensional photonic crystal. Photonic crystals have become powerful tools for confining light in two or three dimensions in devices, including optical fibers, diode lasers, and light-emitting diodes (LEDs), sensors, optical buffers, optical cavities, and switches. Photonic crystals have been discovered in nature and synthetic types are in development for potential mass-market applications including brighter paints and high-efficiency LED illumination sources.
The photonic-crystal concept
Yablonovitch, now at the University of California at Berkeley (Berkeley, CA), says the idea of the photonic crystal grew from his efforts to control spontaneous emission with a pair of closely spaced dielectric mirrors. Seeking to block light from leaking sideways between the mirrors, he proposed dielectric confinement around the edges, leading to proposals for 1-, 2-, and 3-dimensional confinement using photonic crystals. The structures consist of alternating thin layers of two dielectric materials with different refractive indexes (see Fig. 1). Like an interference filter, the layers reflect a resonant band and transmit other wavelengths.In his textbook, John Joannopolis of the Massachusetts Institute of Technology (MIT; Cambridge, MA) calls the resonantly reflected wavelengths a “photonic bandgap,” analogous to the electronic bandgap in a solid. The energy of the photonic bandgap depends on the thicknesses of the layers and their refractive indices. Like electrons, photons can’t propagate through a solid if their energies fall within the bandgap. In most applications, the photonic crystal confines light within a material lacking a photonic bandgap at the same energy, so the light can propagate through it.
Photonic-crystal fibers
One of the first successful applications of photonic crystals was in “holey” or microstructured fibers. Glass tubes are stacked into a bundle, surrounding either a void or a solid glass rod, then heated and drawn into fibers. Long, thin holes run along the length of the fiber, forming a photonic-crystal cladding surrounding a solid or hollow core.
Solid-core photonic-crystal fibers resemble conventional fibers, with the holes reducing the refractive index of the photonic-crystal layer so it acts like a conventional low-index cladding. Hollow-core fibers are fundamentally different, depending on the photonic-crystal effect to trap wavelengths in the photonic bandgap inside the lower-index core region, something otherwise impossible. At one time different terms were used for the two types, with hollow-core fibers called “photonic-bandgap fibers” and solid-core fibers called “photonic-crystal fibers,” but that distinction is often lost today.
Both solid- and hollow-core fibers can be made with otherwise-unobtainable properties. Solid-core fibers can guide light in a single mode in cores larger or smaller than is possible in conventional fibers. Shrinking the core can increase fiber nonlinearity for applications such as supercontinuum generation. Expanding the single mode to fill a larger volume can decrease nonlinearity for applications such as fiber lasers. Hollow-core fibers can be filled with hydrogen for Raman generation, or can be made with special dispersion properties.3, 4 In the long term, hollow-core fibers can offer lower attenuation than solid-core fibers, at least at some wavelengths.
New ideas continue to emerge, such as grading the size of the holes in the photonic-crystal cladding of a hollow-core fiber. Holes with uniform size produce a sharp resonance at one wavelength, with a large fiber dispersion in adjacent bands. To limit the dispersion, Julia Skibina of Saratov State University (Saratov, Russia) and colleagues fabricated the photonic-crystal cladding with holes that increased in size outward (see figure at top of page). This made each layer resonate at a different wavelength, expanding the transmission band and reducing the dispersion, although at some cost in increased attenuation.5
Photonic-crystal lasers and cavities
The extremely high Q factors possible in photonic-crystal cavities makes them attractive for lasers (see www.laserfocusworld.com/articles/308745). The active laser medium occupies a small volume, and like the solid core of a photonic-crystal fiber acts as a “defect” within the bulk photonic crystal. The small size and the ability of the photonic crystal to control spontaneous emission from the active medium allow very low threshold and very fast direct modulation of the laser.
Early lasers were optically pumped and emitted in the near infrared, but progress has been rapid. A year ago, Susumu Nodo’s group at Kyoto University (Kyoto, Japan) reached 406 nm by electrically pumping a photonic-crystal surface-emitting laser (PCSEL).6 They fabricated a gallium nitride (GaN) photonic crystal between the aluminum gallium nitride (AlGaN) cladding layer and the indium gallium nitride (InGaN) quantum well (see Fig. 2).Late last year, Kanna Aoki of the University of Tokyo (Tokyo, Japan) reported another significant achievement–embedding a quantum dot inside a 3-D photonic-crystal cavity to fully confine both electrons and photons.7 A quantum dot coupled its 1.5 mm emission into resonant moves of a 17-layer “woodpile” photonic crystal. The 3-D structure is complex to make, but they say it offers more complete control over the emitted light than a 2-D photonic crystal.
Photonic crystals can also improve LED output efficiency. Several years ago, MIT researchers showed that they could increase output in the vertical direction by a factor of six by fabricating a 2-D photonic crystal within the upper cladding layer of a quantum-well LED.8 Lead author Alexei Erchak is now chief technology officer at Luminus Devices (Billerica, MA), which uses photonic-crystal structures to develop GaN LEDs for illumination. A less-complex photonic-crystal design, which etches simple periodic gratings onto the surface of InGaN/GaN quantum-well LEDs, can increase output efficiency more than 90%, a team from National Taiwan University (Taipei, Republic of China/Taiwan) reported at last year’s CLEO.9
3-D Fabrication
Stacking glass tubes together and drawing them into fibers offers a straightforward way to make photonic-crystal fibers. Three-dimensional photonic crystals have posed a more formidable challenge. Woodpile-type structures require laboriously stacking layers to build a thick object, with removing material a particularly difficult challenge. The process works in the laboratory, but commercial production is a challenge.
One alternative is looking good, says Yablonovitch. “We’re very close to having a way to make inexpensive 3-D photonic crystals, using a holographic writing approach,” developed by Andrew Turberfield at the University of Oxford (Oxford, England). Multiple laser beams expose a photoresist-type polymer, and the unexposed material is removed to leave a three-dimensional lattice. Then the lattice is filled with titanium dioxide and the exposed photoresist is etched away to create holes.10
An intriguing near-term potential application is to use small TiO2 (titanium dioxide) photonic crystals as paint pigments. “Just three periods gives you a lot of scattering,” says Yablonovitch. That would make the paint much brighter than conventional paints. Similarly, TiO2 photonic crystals could boost the brightness of paper. And don’t expect it to stop there. “You can be sure this will just keep going; it’s a totally painless way to make photonic crystals,” Yablonovitch says.
Outlook
The potential of photonic crystals is tremendous. Browsing through the long list of CLEO/QELS papers shows a wide range of applications. Jelana Vukovic’s group at Stanford University (Stanford, CA) coupled light from a colloidal quantum-dot film to a silicon photonic-crystal cavity, and is investigating prospects for a laser.11 Other papers in the regular session reported photonic-crystal switches, sensors, and other devices.
In the postdeadline session, NTT Basic Research Laboratories (Kanagawa, Japan) described Q-switching sub-60 ps pulses from an ultrahigh-Q photonic-crystal cavity by modulating the cavity Q. They say that development could lead to “a sophisticated photonic memory or a wavelength converter that operates even at the single-photon level.”12
Although the promise is large, so are the challenges. Fabricating complex photonic-crystal devices remains a serious issue. Getting light out of the photonic crystal can be tricky. Some applications may never work, but others look promising.
References
- E. Yablonovitch, Phys. Rev. Lett. 58, p. 2059 (1987).
- J. Joannopolis et al., Photonic Crystals: Molding the Flow of Light, 2nd ed. (Princeton University Press, Princeton, NJ, 2008).
- F. Couny, F. Benabid, and P.S. Light, CLEO 08, paper CTuL4.
- J. Fekete et al., CLEO 08, paper CTuV3.
- J. Skibina et al., Nature Photonics 2, p. 579 (November 2008).
- H. Matsubara et al., Science 319, p. 445 (Jan. 25, 2008).
- K. Aoki et al., Nature Photonics 2, p. 688, (November 2008).
- A.A. Erchak et al., Appl. Phys. Lett. 78, pp. 563, January 2001.
- Cheng-Yen Chen et al., CLEO 2008, paper CMKK5.
- M. Campbell et al., Nature 404, p. 53 (March 2, 2000).
- B. Ellis et al., CLEO-QELS, San Jose, CA, May 2008, paper CTuW5.
- T. Tanabe et al., CLEO/QELS 2008, postdeadline paper QPDB1.
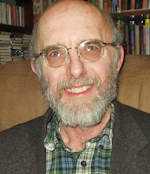
Jeff Hecht | Contributing Editor
Jeff Hecht is a regular contributing editor to Laser Focus World and has been covering the laser industry for 35 years. A prolific book author, Jeff's published works include “Understanding Fiber Optics,” “Understanding Lasers,” “The Laser Guidebook,” and “Beam Weapons: The Next Arms Race.” He also has written books on the histories of lasers and fiber optics, including “City of Light: The Story of Fiber Optics,” and “Beam: The Race to Make the Laser.” Find out more at jeffhecht.com.