ULTRA-HIGH-POWER LASERS: Wavefront correction is achieved for 200 TW ALLS
Researchers from the University of Québec (INRS-EMT, Varennes, Québec, Canada), Moscow State Open University, and Night N (both in Moscow, Russia) have used large-aperture adaptive optics to implement laser-beam wavefront correction on the 200 TW laser system at the Advanced Laser Light Source (ALLS) in Varennes near Montreal.1 To the researchers’ knowledge, this is the first ultrahigh-power laser that simultaneously combines ultrahigh intensity, high contrast ratio, and high repetition rate for practical applications in laser-matter interaction studies.
Although certain laser-matter applications—high-energy particle acceleration and bright-x-ray-source studies—can be performed with lasers having peak intensities in the 1018 to 1019 W/cm2 range, applications such as proton generation using ultrathin solid foil targets and the development of high-flux laser-based hard-x-ray sources used for medical imaging require high-energy laser sources in the 1020 W/cm2 intensity range, as well as both high peak and average power with an ultraclean temporal shape.
To obtain such high intensity, a small focal spot for the laser is typically required. Today’s high-power Ti:sapphire laser systems can routinely achieve pulses with a 25 fs duration, but increasing the laser energy induces thermal heating effects in the amplification crystals that deform the laser wavefront. Focusing the laser with an off-axis parabola can avoid nonlinear effects and reduce wavefront distortion; however, these optics are difficult and expensive to machine.
Despite these challenges, researchers have implemented adaptive-optics systems using deformable mirrors on several Ti:sapphire systems with laser intensities up to 1022 W/cm2. Unfortunately, the short focal length of 0.6 of these systems and their 0.1 Hz repetition rate is not suitable for many applications. And although nearly diffraction-limited focusing was achieved with the 100 TW laser system at the Japan Atomic Energy Research Institute (JAERI) with a 1020 W/cm2 intensity for a 23 fs laser pulse duration, this implementation had a poor laser-pulse contrast ratio (defined as the ratio of the peak pulse power to the background noise of the laser).
Adaptive optics
To combat these drawbacks on the 200 TW ALLS, wavefront characterization and beam correction are achieved using a wavefront sensor coupled to a deformable mirror and focusing optics with a focal length of 3. The ALLS (built by Amplitude Technologies near Paris, France) uses Ti:sapphire technology and chirped-pulse amplification (see also www.laserfocusworld.com/articles/318551). With a center wavelength of 800 nm and a 10 Hz laser repetition rate, the ALLS consists of an oscillator, a booster with a solid-state saturable absorber to increase pulse contrast, a grating-based stretcher to increase pulse duration up to 350 ps, a regenerative amplifier, and a power-amplification stage pumped with 14 Nd:YAG lasers for 16 J of total pump energy.
Initially, an 18 fs pulse with a 100 nm spectral bandwidth is produced by the oscillator. An acousto-optic programmable dispersion filter (AOPDF) is placed before the stretcher to control the spectral phase, and a second AOPDF is placed in the regenerative cavity to control the spectral amplitude of the pulse, enabling a 55-nm-bandwidth full-width-half-maximum pulse after compression with 25 fs pulse duration and 150 TW of peak power.
Measurements using a high-dynamic-range third-order cross-correlator yield a laser pulse contrast ratio of better than 109. This parameter is crucial for applications involving interactions of the laser beam with high-density solid targets. To prevent changes in beam divergence due to thermal lens effects and pulse wavefront distortions caused by thermal loading, cryogenic units cool the Ti:sapphire crystal down to -80°C in the last two amplification stages.
Optimized laser focusing is achieved with a deformable mirror composed of 48 piezoelectric-ceramic electrodes controlled by high voltage. Accuracy of the wavefront measurement system is λ/20. The wavefront is measured by transposing the beam to a wavefront sensor using a reducing telescope. The Shack-Hartmann wavefront sensor allows reconstruction of the wavefront phase map of the beam. Beam wavefront and focal-spot measurements were made both before and after wavefront correction by the deformable mirror (see figure).
Without applied voltage to the deformable mirror, the root-mean-squared (rms) wavefront value is 0.471λ and the laser spot size is 8.6 × 14.8 µm2 at 1/e2 of the laser’s peak intensity of 1.2 × 1020 W/cm2. After applying wavefront correction, RMS wavefront value is significantly reduced to 0.063λ and 1/e2 spot size is also reduced to 7.0 × 7.8 µm2 with a corresponding peak intensity of 3 × 1020 W/cm2 (more than a factor of two improvement compared to the uncorrected wavefront result).
Researcher Sylvain Fourmaux from the University of Québec says, “Our objective is now to demonstrate that such a laser system can be used for practical implementation of high-flux, hard-x-ray sources or proton beams.”
REFERENCE
- S. Fourmaux, et al., Optics Exp. 16, 11987 (Aug. 4, 2008).
About the Author
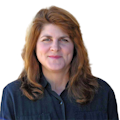
Gail Overton
Senior Editor (2004-2020)
Gail has more than 30 years of engineering, marketing, product management, and editorial experience in the photonics and optical communications industry. Before joining the staff at Laser Focus World in 2004, she held many product management and product marketing roles in the fiber-optics industry, most notably at Hughes (El Segundo, CA), GTE Labs (Waltham, MA), Corning (Corning, NY), Photon Kinetics (Beaverton, OR), and Newport Corporation (Irvine, CA). During her marketing career, Gail published articles in WDM Solutions and Sensors magazine and traveled internationally to conduct product and sales training. Gail received her BS degree in physics, with an emphasis in optics, from San Diego State University in San Diego, CA in May 1986.